Compound is commonly used to control blooms of cyanobacteria in fish and shrimp ponds
In this article, we will discuss the applications of copper sulfate in aquaculture, special precautions for its use, the development of copper resistance by cyanobacteria and alternative products.
Pentahydrated copper sulfate (CuSO4.5H2O), referred to as CS from here on, is the most commonly used form of copper for algae control in aquaculture due to its high solubility in water, availability and low cost. CS is a blue crystalline solid at room temperature, containing copper ions (Cu+2) and sulfate ions (SO4-2) and five water molecules (H2O) in its structure, hence the term “pentahydrated.” Copper sulfate is highly soluble in water, and its solubility decreases as water temperature decreases and water pH increases. Another highly soluble copper source in water is dihydrated copper chloride (CuCl2⋅2H2O), consisting of copper and chloride ions (Cl−), and two water molecules, in the form of green crystals. In times of CS scarcity, some producers have instead used copper oxide (CuO), available as dark crystals ranging from black to dark gray or dark reddish-brown; copper oxide is poorly soluble in water and should not be used.
Applications of copper sulfate in aquaculture
Copper sulfate is mostly used to control cyanobacteria (blue-green algae) in pond water. Dense blooms of cyanobacteria cause night-time depletion of oxygen and increased carbon dioxide concentrations, as well as high pH fluctuation during the day. Cyanobacteria also produce substances like geosmin (GSM) and 2-methylisoborneol (MIB) that can impart off-flavor in cultured fish and shrimp. Cyanobacteria also produce toxic microcystins (MCs) which are hepatotoxic (chemical-driven liver damage) and in high concentrations in water, cause discomfort, impair performance, reduce immunity, and can even cause acute intoxications and death to fish and shrimp. Hence, it is crucial to control cyanobacterial blooms in aquaculture ponds.
Copper sulfate is also effective and economical in the control of infections by some parasites, fungi (Saprolegnia sp.), and external bacteria such as Flavobacterium columnare. CS is also used to control filamentous algae, macrophytes, and aquatic mollusks. Its low cost and effective low doses (between 0.8 and 2.0 g CS/m2) make CS one of the first options for controlling algae and for treating parasites and other potential fish diseases in large ponds.
Considerations on copper sulfate use in fish ponds
Although CS is not a dangerous product to handle, it’s advisable to wear gloves, protective goggles and long-sleeved clothing when handling the product and its solutions. It’s also important to store and keep the product out of the reach of children and animals. A copper sulfate solution is usually sprayed over the entire pond surface or applied in front of the water flow promoted by aerators, which favor the distribution of the chemical solution throughout the pond.
Copper sulfate is not very selective for a specific type of algae. It affects both cyanobacteria and green algae (chlorophytes) indiscriminately. However, some studies have shown that the CS concentrations required to impact green algae are at least five times higher than for cyanobacteria. This is useful, as copper sulfate applied at the lowest effective doses to control cyanobacteria will spare green algae, allowing them a chance to get established and overcome cyanobacteria populations in numbers and biomass. It also improves water quality and helps reduce problems with “off-flavors” in fish and shrimp flesh.
The toxicity of copper (Cu+2) to microalgae, parasites, and other aquatic organisms is regulated by water chemical characteristics, especially pH, alkalinity (bicarbonate, carbonate, and hydroxyl ions), and water hardness (calcium ions, Ca+2; and magnesium ions, Mg+2), being more toxic in acid and low alkalinity and low hardness waters. So, it is important for the aquafarmer to understand the chemical conditions of pond water at the time of application.
Some fish and shrimp species may be more susceptible to CS than others. Therefore, farmers must know the tolerance of the species they work with and carry out bioassays with a small number of animals of any new species to determine effective and safe doses of CS to be used.
Copper sulfate applications in tanks and ponds with green water can result in dissolved oxygen deficits due to microalgae die-offs, even if the application was made for a different purpose, such as parasite control. Indirectly, microalgae will be affected. Therefore, it’s essential to monitor water oxygen levels and have mechanical aerators ready for use if necessary.
The decay and decomposition of dead cyanobacteria after CS treatments further increases the concentration of geosmin (GEO), 2-methylisoborneol (MIB) and microcystins (MCs) in water. Therefore, CS treatments of aquaculture ponds carry some risks, and may augment the intensity of “off-flavor,” making fish and shrimp unsuitable for sale and consumption.
Fish such as Nile tilapia cultured in ponds can ingest large amounts of cyanobacteria containing GEO, MIB and MCs daily. Thus, it’s essential to perform preventive and gradual control of cyanobacteria and not wait for any populations to become strongly established in a pond before taking appropriate action.
After CS application, it is recommended to not discharge pond water for the next two to three weeks. This allows enough time for most copper ions (Cu+2) to be complexed by carbonates, hydroxyls, and phosphates present in the water and to settle on the pond bottom soil. Once deposited in the sediments, copper becomes trapped in the clay fraction of the soil. Also, when seining a pond (like during a sampling or harvest) do not exchange/discharge any pond water to prevent effluents loaded with solids (suspended soil, algae and organic matter) being released to surrounding natural waters. This is an effective practice to minimize the discharge of copper, other nutrients, silt and clay, and organic matter into rivers and other natural aquatic environments.
Water chemistry and CS treatments
Water chemistry influences the efficacy of copper sulfate treatments. Dissociation of copper ions is faster in water of low pH (more acidic). In intensive aquaculture ponds, the water pH is often lower (6.0 to 7.5) near the sunset, and it rises throughout the day due to the photosynthesis of microalgae, often reaching values above 9.0. Hence, CS treatments are more effective when applied in the early morning hours. Total alkalinity (TA) is the sum of bases in the water (mainly bicarbonate, HCO3– ; carbonate, CO3=; and hydroxyl, OH–). These bases react with free copper ions (Cu+2) to form insoluble complexes, reducing the effect of copper on targeted algae and pathogens. For this reason, the CS dosage needs to be higher with increasing total alkalinity.
The equation CS (g/m3) = TA/100 is suggested to estimate the proper dose of CS, ensuring an effective dosage with a lower risk for the cultured species. For example, with a TA of 50 mg CaCO3/L, a safe CS dose would be 50/100 = 0.5 grams per cubic meter. With a TA of 100 mg CaCO3/L, the safe CS dose can go up to 1.0 grams per cubic meter. However, when the safe dose estimated from the equation is below 0.5 mg per liter, it might not be sufficient for controlling microalgae or pathogens. Usually, doses of at least 0.8 to 1.0 grams of copper sulfate per cubic meter are necessary for efficient cyanobacteria and pathogens control. Smaller doses, even at lower total alkalinity, are often ineffective. Therefore, it is essential to know the tolerance of the cultured species to copper to establish an effective and safe dose of copper sulfate application.
In large ponds, the CS doses applied for controlling parasites and external bacteria are the same safe doses recommended for cyanobacteria control (around 1 gram CS per cubic meter). In cases where TA exceeds 100 mg CaCO3 per liter, it is recommended to use the TA/100 equation to determine the dose. If TA is 200, the CS dose to be applied should be close to 2 grams per cubic meter. For some parasites (e.g, dinoflagellates, protozoa like ichthyophthiriasis), as well as for the control of fungi and external bacteria, it might be necessary to make three to four applications at intervals of three to five days. Always consult an experienced professional to define the most suitable protocol for CS and other therapeutic products used in your farm.
The proper use of the Secchi disk to assess water transparency is very important when calculating proper copper sulfate doses.
Microalgae (cyanobacteria) density in pond water also influences CS efficacy. Generally, larger doses of CS are needed in excessively green waters (with large amounts of algae). When farmers decide to apply CS for cyanobacteria control, the pond water often has low transparency (15 to 25 cm using a Secchi disk). At these transparency values, CS doses around 0.8 to 1.0 grams per cubic meter are sufficient for microalgae control. However, with water transparencies below 10 cm, CS doses above 1.5 to 2 grams per cubic meter may be necessary.
Copper tolerance and mutational capacity of cyanobacteria
Cyanobacteria have various mechanisms to increase their tolerance and survive with excess Cu+2 ions and other metallic ions and toxic substances in water. One mechanism is the secretion of proteins (exoproteins) into a biofilm they form on the surface of their cells. The exoproteins trap metallic ions and decrease the concentration of free ions in the environment, preventing them from entering the cells in excess.
This provides particular protection to cyanobacteria from copper, especially when their cells group together in colonies. Another is the production of specific proteins that bind to metallic ions (metallothioneins) inside the cells, reducing the toxic effects of Cu+2 ions that have been absorbed. Also, they have polyphosphate granules inside their cells, which can bind to metallic ions like Cu+2, or active transport mechanisms for Cu+2 ions out of the cell. Finally, increased production and activity of enzymes like catalase (CAT) and superoxide dismutase (SOD) in response to environmental stress caused by the presence of metallic ions like copper. These enzymes neutralize reactive oxygen species (ROS) harmful to cells; ROS are abundantly generated in the presence of oxidative ions like copper.
Cyanobacteria frequently exposed to copper can undergo genetic changes (mutations) in genes responsible for the coding, production, or expression of exoproteins, metallothioneins and the enzymes CAT and SOD. Researchers have reported spontaneous mutations that occurred in cells of Microcystis aeruginosa after exposure to high doses of copper sulfate. These mutants were able to thrive in environments with copper concentrations above 0.37 mg Cu+2 per liter, equivalent to 1.5 mg of CS per liter. Another study found 1.8 mutants for every 1 million cell divisions. Cyanobacteria in pond water can easily exceed 2 billion cells per liter, and more than 3,600 mutant cells per liter can arise with each cell division. Thus, with frequent copper applications in ponds and constant elimination of original cells, a predominantly copper-resistant cyanobacteria population could establish itself in a few generations.
In a study under controlled laboratory conditions, it was shown that in a few culture cycles the tolerance of the cyanobacterium Microcystis aeruginosa to copper increased 4 to 12 times, reaching tolerance levels near 0.8 mg Cu+2 per liter (equivalent to 3.2 mg CS per liter). A specific population of Microcystis tolerated up to 1.9 mg Cu+2 per liter (equivalent to 7.5 mg CS per liter). And in a practical trial with water rich in Microcystis aeruginosa collected from a commercial fish farming pond, the commonly applied dose of 1 mg CS per liter did not reduce the cyanobacteria count compared to the control. This suggests that the Microcystis population in that farm have developed resistance to copper sulfate due to its repeated use. In the same trial, treatments with hydrogen peroxide at 15 and 30 ppm were very effective in cyanobacteria control (Fig. 1). Several other studies indicate that hydrogen peroxide at doses between 5 and 15 ppm selectively controls cyanobacteria in fish farming ponds.
Lethal and safe doses of copper for tilapia and marine shrimp
For Nile tilapia (Oreochromis niloticus), the lethal concentration of copper ions (Cu+2) that kills half of the animals in 96 hours (LC50-96h) was determined for fry (weight of 10 mg, length of 7 mm) at 0.05 mg Cu+2 per liter using copper chloride (0.125 mg CuCl2 per liter) and 0.12 mg Cu+2 per liter using copper nitrate (0.457 mg per liter Cu(NO3)2). For 3-gram juveniles, in water with pH 8.2 and total alkalinity of 196 ppm, the LC50-96h was estimated at 7.94 mg Cu+2 per liter with copper sulfate (31.2 mg per liter CuSO4.5H2O). For 90-day-old tilapia juveniles, in water with pH 7.25 and total hardness of 255 ppm, the LC50-96h was estimated at 35 mg Cu+2 per liter with copper sulfate (100 mg per liter CuSO4.5H2O). A concentration equivalent to 5 percent of LC50-96h is generally considered as safe.
For Pacific white shrimp (Litopenaeus vannamei), copper tolerance was evaluated for different life stages in water with 15 ppt salinity and pH of 7.5 to 8.0. Copper tolerance increased with developmental stages. For nauplii, the most sensitive phase, the LC50-24h ranged from 9.5 to 15 micrograms Cu+2 per liter (or parts per billion). In the zoea stages, the LC50-24h varied between 16 and 32 µg Cu+2 per liter. For mysis stages 1, 2 and 3, the average LC50-48h were 85, 200 and 370 micrograms Cu+2 per liter, respectively. For PL5 (five-day-old postlarvae), the LC50-96h ranged from 0.64 to 1.5 mg Cu+2 per liter, and 1.1 to 2.3 mg Cu+2 per liter for PL10-15. For 9-gram shrimp, the LC50-96h ranged from 4 to 3.1 mg Cu+2 per liter. In a full-strength seawater study, the LC50-96h for 2 g shrimp was 35 mg Cu+2 per liter.
Shrimp survival was assessed under continuous exposure to copper concentrations of 10, 5, 2.5, and 1 percent of the LC50-96h of 35 mg Cu+2 per liter. Total mortality occurred within 6 weeks in shrimp continuously exposed to concentrations equal to or higher than 2.5 percent of LC50-96h, i.e., 0.88 mg Cu+2 per liter (equivalent to 3.6 mg CS per liter). Shrimp continuously exposed to 1 percent of LC50-96h (0.35 mg Cu+2 per liter or 1.4 mg CS per liter) had 100 percent survival. These two studies had contrasting results regarding the CS tolerance of L. vannamei juveniles, which seems to be much higher at higher water salinity. Natural waters with higher salinity (such as seawater) generally have higher alkalinity and total hardness than low-salinity waters. Cyanobacteria problems in shrimp farms occur in low salinity waters, where hardness and alkalinity values are much lower. Thus, effective CS doses of 0.8 to 1.2 grams per cubic meter, equivalent to 0.2 to 0.3 mg Cu+2 per liter, initially reach copper concentrations between 10 and 30 percent of the LC50-96h determined for L. vannamei at 15 ppt salinity (1.1 and 3.1 mg per liter Cu+2 per liter).
Therefore, for L. vannamei farming in low salinity waters it is important to check the alkalinity and hardness of pond water to be treated with CS. In fact, before applying the product in an entire pond, it is advisable to conduct a preliminary test of the CS target dose with a few animals in a small container (1-cubic-meter tank, for example) with the same pond water to be treated.
Algaecides and parasiticides alternatives to copper sulfate
CS can be used at low dose levels and is inexpensive and easy to procure. It is effective for the control of algae, some parasites and other potential pathogens. However, its recurrent use in aquaculture farms favors the establishment of Cu+2 resistant cyanobacteria, and, possibly, more tolerant parasites and pathogens. This is already happening on many farms, requiring the application of higher CS doses. The effective CS doses used to control cyanobacteria and pathogens are already very close to the toxic concentrations of copper to some fish and shrimp species. A further increase in doses will increase the risk of using CS. In addition, increased levels of copper in pond sediments and effluents should be expected with the continuous use of CS. This raises environmental concerns regarding the possible deleterious effects of copper on non-target aquatic organisms in natural waters receiving aquaculture effluents. Consequently, it is necessary to find alternatives to CS for the control of cyanobacteria and fish pathogens in large ponds.
Diuron (diclofenil-dimethyl-urea) is an herbicide that has selective action against cyanobacteria while preserving other microalgae. Diuron was registered in the USA for controlling algal blooms in fish ponds and has a very attractive cost, making it even more economical per application than CS. Its effective dose is around 0.01 mg of active ingredient (AI) per liter. In practice, 0.01 grams AI per square meter or 100 grams AI per hectare, for a product cost close to (Brazilian reais) R$ 7.00 (US$1.42)/ha per application, compared to R$ 350.00 (US$71)/ha for CS at 1 gram CS per square meter or 10 kg CS per hectare.
However, similar to CS, Diuron also has a high environmental persistence, and its continuous use can affect non-target organisms. Concerns also exist about its accumulation in fish flesh after repeated applications in ponds, and its residues can also impact aquatic communities in water bodies receiving effluents from aquaculture farms. Like CS, the repeated use of Diuron can result in the development of cyanobacteria populations resistant to this herbicide, as demonstrated in several studies, making the product less effective over time. Even though it is approved by the U.S. Environmental Protection Agency (EPA), there are indications that Diuron has carcinogenic effects and other risks to human health, and the environmental and human health issues will continue to put pressure on the use of Diuron and other chemicals in aquaculture.
Hydrogen Peroxide (HP, H2O2) can replace copper sulfate. HP is very effective in reducing cyanobacteria in aquaculture ponds. Concentrated liquid formulations with 30 or 50 percent HP and sodium percarbonate (known as “powdered oxygen”) are approved in the USA and European countries for applications in aquaculture. HP is also approved to reduce cyanobacteria, and odorous compounds in water entering treatment plants for human consumption, as well as to reduce odors (caused by hydrogen sulfide, methane, and other volatile compounds), cyanobacteria and pathogenic microorganisms in effluents from sewage treatment plants. HP reacts rapidly with microalgae cells and suspended organic material, decomposing into oxygen and water without leaving toxic residues in the environment. An advantage over CS and other products to control cyanobacteria is that HP simultaneously oxidizes microcystins, geosmin (GEO), 2-methylisoborneol (MIB), and probably other toxic and odorous compounds in the water.
Thus, HP helps reduce the intensity of “off-flavor” and the risk of fish intoxication. HP is already widely used to treat fungi (Saprolegnia sp.) and bacterial infections in fish eggs (trout, salmon, catfish, and tilapia) and is also effective in the treatments of parasites (protozoa, dinoflagellates, and monogeneans), fungi and external bacteria in large ponds, as well as in concentrated short baths. Consequently, hydrogen peroxide is an alternative to CS for most of its applications in aquaculture, with the advantage of not leaving residues in fish or the environment. Doses of HP from 4 to 15 ppm (4 to 15 g H2O2 per cubic meter) are effective in controlling cyanobacteria in fish ponds and are very safe for most species of warmwater fish. We have observed effective results with the use of HP at doses of 4 to 8 ppm (Fig. 2). A 4-ppm hydrogen peroxide dose has a product cost per application similar to that of 1 ppm CS.
Final considerations
Although CS is effective and economical in controlling algae and parasites, its repeated use and the need for increased doses can present risks to aquatic ecosystems. As it does not have a selective action, it ends up affecting other beneficial organisms. And the accumulation of copper in sediments and its potential to contaminate bodies of water raise concerns about the environmental impact of the use of copper sulfate.
Given the increasing demand for the adoption of more sustainable production practices, reducing environmental impacts and improving food security, the aquaculture sector must be sensitive and open to the need for alternative products or management practices that can replace the use of chemicals such as copper sulfate due to its potential impacts.
Now that you've reached the end of the article ...
… please consider supporting GSA’s mission to advance responsible seafood practices through education, advocacy and third-party assurances. The Advocate aims to document the evolution of responsible seafood practices and share the expansive knowledge of our vast network of contributors.
By becoming a Global Seafood Alliance member, you’re ensuring that all of the pre-competitive work we do through member benefits, resources and events can continue. Individual membership costs just $50 a year.
Not a GSA member? Join us.
Author
-
Fernando Kubitza, Ph.D.
Jundiaí, São Paulo, Brazil
Dr. Fernando Kubitza is a Brazilian aquaculture specialist and director of Acqua Imagem, www.acquaimagem.com.br, a leading company for 25 years on aquaculture projects, training and technical support in Brazil.[114,98,46,109,111,99,46,109,101,103,97,109,105,97,117,113,99,97,64,111,100,110,97,110,114,101,102]
Tagged With
Related Posts
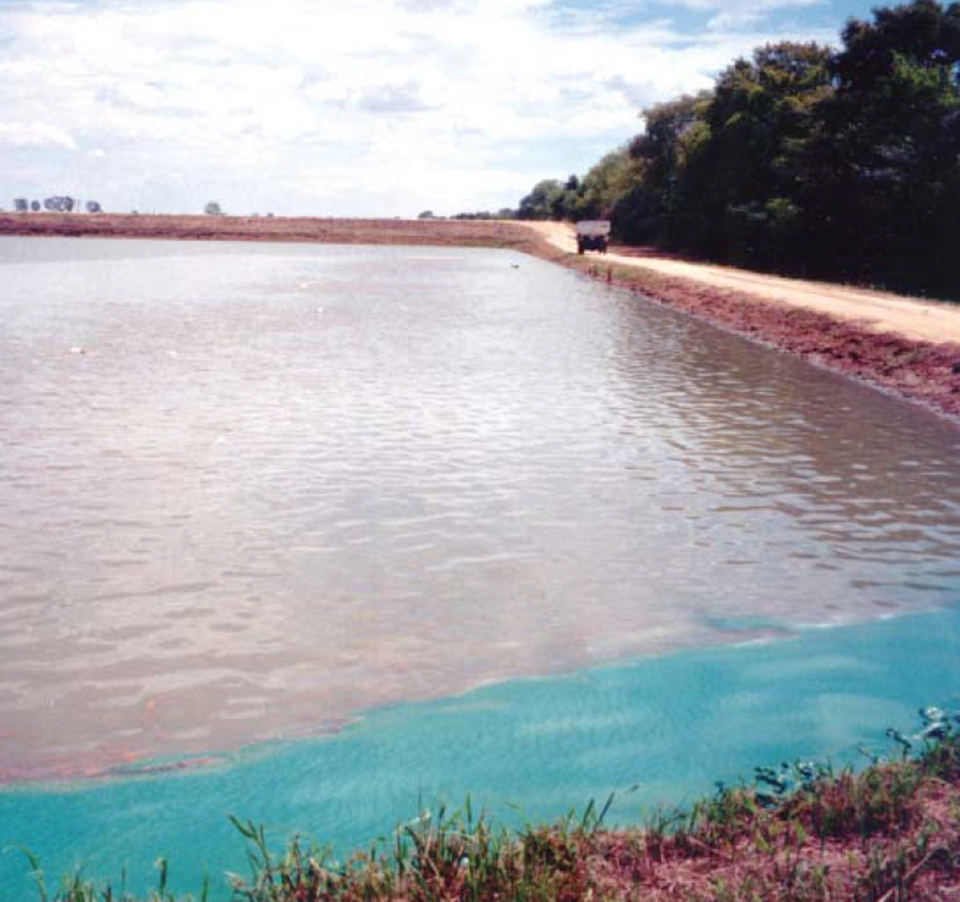
Health & Welfare
Off-flavors in channel catfish managed with low-dose applications of copper sulfate
Off-flavors in farmed fish cost the U.S. catfish industry millions but their prevalence can be greatly reduced by copper sulfate treatment of pond water.
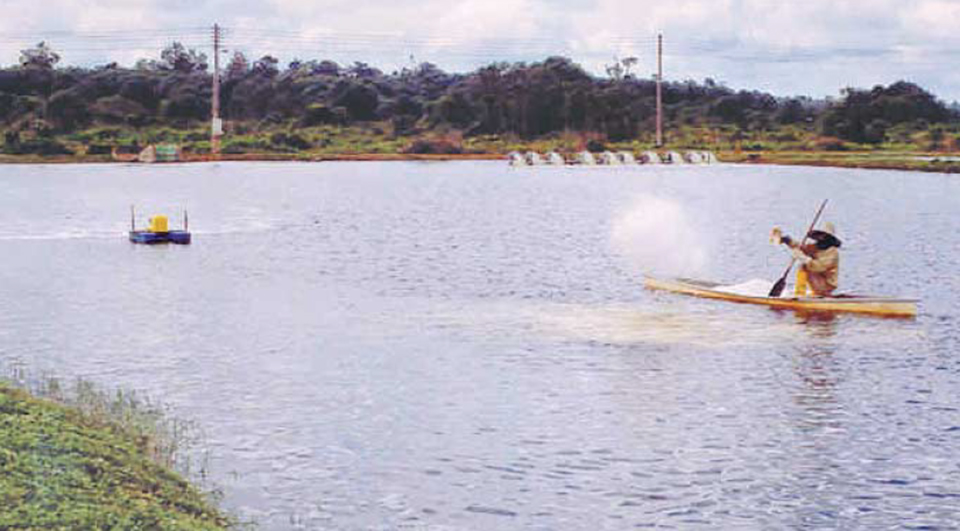
Responsibility
Chemical fertilizers in pond aquaculture
Chemical fertilizers are frequently used in pond aquaculture to stimulate phytoplankton productivity and enhance the availability of natural food organisms.
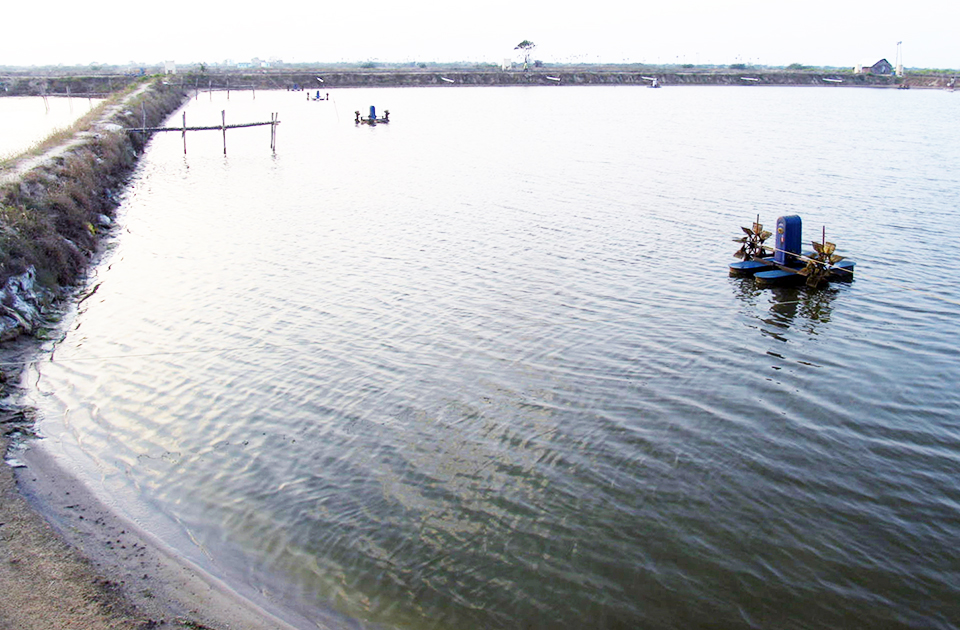
Responsibility
Examining copper use in aquaculture
Copper is used for control of the blue-green algae responsible for off-flavors in aquaculture animals, treating diseases and parasites, and avoiding cage net fouling. Although copper is an essential nutrient for plants and animals, an excess can negatively affect the environment and human health.
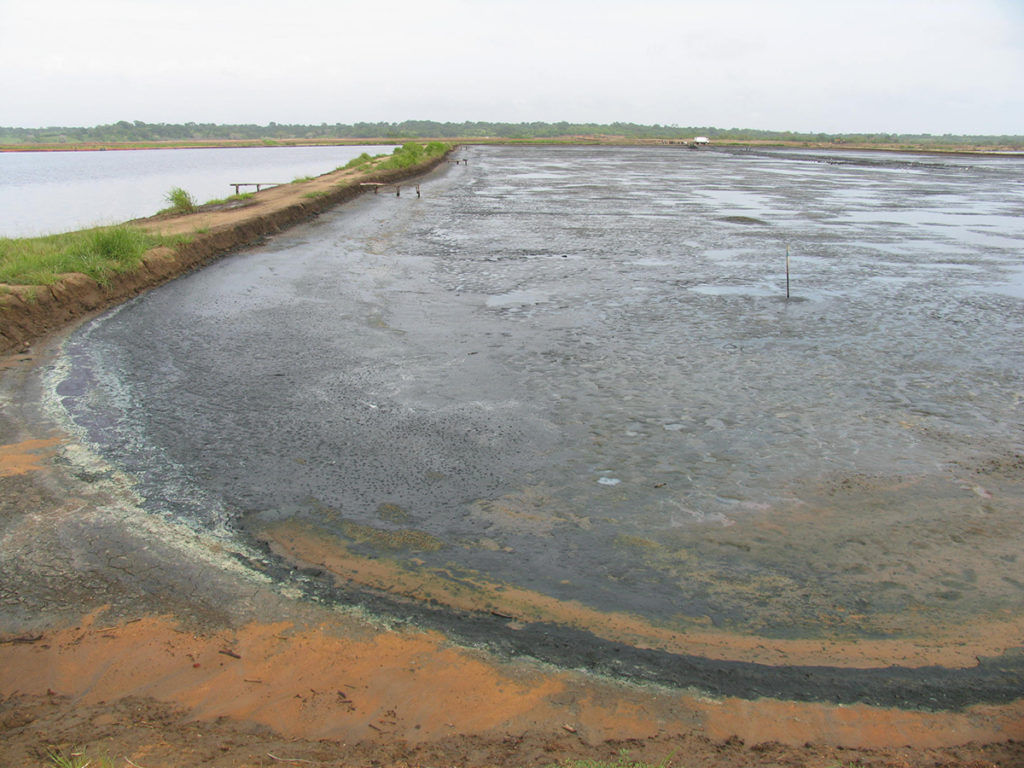
Responsibility
Shrimp pond preparation crucial for production, disease prevention
Preventing diseases is a major concern in shrimp farming. Pond preparation focuses on avoiding the carryover of disease organisms from one crop to the next.