Life cycle assessment useful in evaluating sustainability
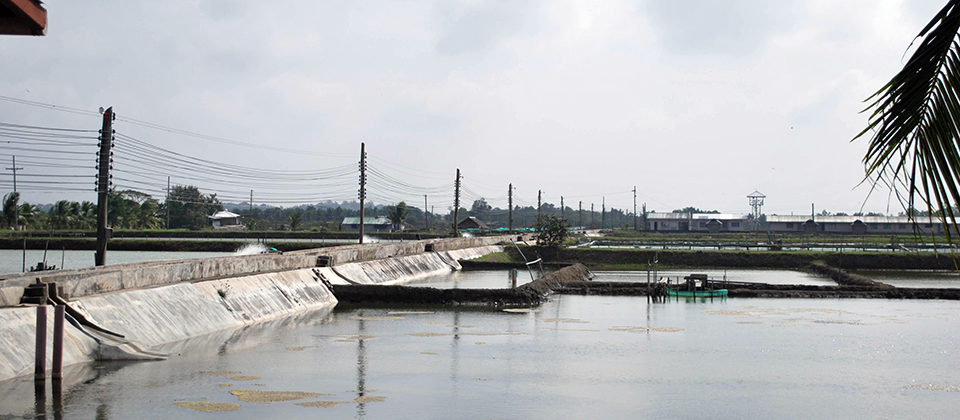
Inexpensive energy is often seen as fundamental for economic growth. It has also enabled rapid improvements in living standards over the last century, thanks to constant withdrawals from the global fossil fuel account.
Food production, in common with other industries, has become addicted to cheap energy. Production, distribution and consumption of food accounts for 20 to 25 percent of the energy consumption in developed countries. The largest energy investments are made in the production of protein-rich produce, such as meat and fish (Table 1).
Henriksson, Energy use in food production systems, Table 1
Edible Produce | MJ kg-1 |
---|
Edible Produce | MJ kg-1 |
---|---|
Beef | 43-64 |
Farmed salmon | 26-48 |
Pangasius | 12-56 |
Global fisheries | 24 |
Tilapia, intensive | 18-27 |
Broiler chickens, U.S. | 15 |
Milkfish | 7-16 |
Wheat | 4 |
Soybeans | 2-3 |
Oysters | < 1 |
Aquaculture energy consumption
Feed production is commonly the major energy-consuming process in finfish and crustacean production systems. Processing accounts for roughly an additional 10 percent of the energy use for most seafood products, while consumption contributes about 20 to 30 percent. For filter feeders, the common energy-intensive stages are transportation and holding of live animals, infrastructure and processing, depending upon final product form and if there is a purification stage.
Transportation accounts for only a few percent of the energy used in the life cycle of farmed salmon, while feed production accounts for over 90 percent. When fresh seafood is transported by air, the emissions from transport become significant.
Energy intensity is not only a good indicator of environmentally unsound systems, but also of systems vulnerable to fluctuations in energy prices. Future increases in energy prices can be expected, as oil will likely become a limited resource over the coming century, and climate change policies are implemented.
A recent report by the United States Joint Forces Command stated: “By 2012, surplus oil production capacity could entirely disappear, and as early as 2015, the shortfall in output could reach nearly 10 million barrels per day.” The food sector will therefore be faced with challenges to keep up supply, especially since the global population is demanding more animal proteins as a result of rapid economic growth in emerging economies.
Life cycle assessment
Aquaculture presents itself as a promising alternative to currently inadequate capture fisheries and inefficient livestock production. This status, however, is highly dependent upon which path the industry takes, as it is still under rapid development.
To guide the industry toward best practices, a thorough evaluation of the sustainability of different aquaculture practices, entailing the whole production chain from extraction of resources to landfill, needs to be carried out. In this process, life cycle assessment can be a highly useful tool.
Life cycle assessment allows evaluations of several environmental impacts to be managed within the same framework, including global warming, water use, land use, depletion of fossil energy, etc. The tool has previously been used to show that closed systems motivated by environmental concerns such as eutrophication, escapes and spread of disease/parasites, can result in other concerns, such as larger energy dependency and greenhouse gas emissions. These trends have also been found for Pangasius farms in Southeast Asia, where increased pumping of water, instead of tidal exchange, has resulted in significantly higher fossil fuel consumption.
Some environmental consequences may be directly related to feed production, and feed often accounts for over 90 percent of the energy use. Both the feed-conversion ratio and feed composition directly affect the environmental impacts of feed-related production. Plant-based ingredients commonly have less impact, while fishmeal, fish oil and livestock processing coproducts such as offal cause greater effects. Lower feed inputs in extensive farming also allow for more energy-efficient production.
Socioeconomic factors
It is also important not to forget socioeconomic factors when comparing systems. If they are not considered, problems will only be shifted rather than resolved. For example, most aquaculture farms still have a high dependency on fishmeal. Peru is the largest producer of fishmeal, while 25 percent of Peruvian infants are still suffering from malnourishment.
Utilizing crop-derived feed inputs not directly available for human consumption should be encouraged, and direct consumption of forage fish should be promoted. Furthermore, a third of the global food production is currently wasted before it reaches consumers. To reduce this fraction, improvements are needed in the logistics and utilization of food, as well as a change in our perceptions of food.
With an increasing portion of aquatic products entering international markets, there is a need for high scrutiny. Mismanaged capture fisheries in Europe and other parts of the world have resulted in increasing imports of seafood from faraway places, much of which is derived from aquaculture. Some of these imports may, however, contribute to ecosystem degradation and pollution. At times, they may also deprive the local poor of essential sources of proteins.
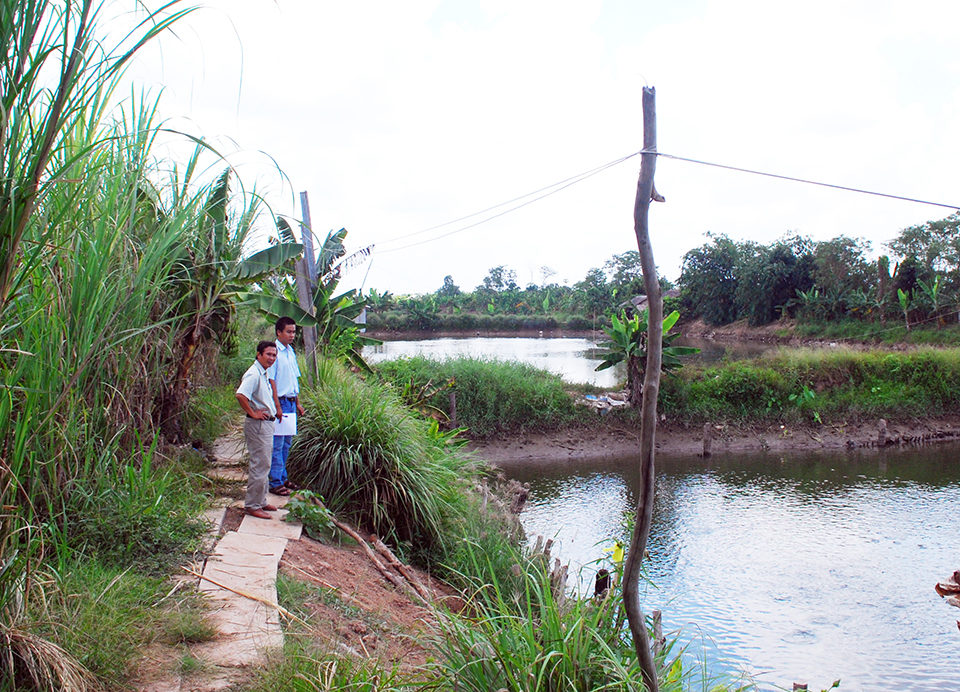
Systems approach
Future sustainability evaluation schemes need to use a broad systems approach to cover all areas of concern. Areas of concern are often interlinked, and it is necessary to consider the true consequences of the whole production chain before making decisions. The aim should be to improve human well-being and equity for all relevant stakeholders and developed aquaculture in the context of other sectors, policies and goals.
A good example of such an approach is presented by the Sustaining Ethical Aquaculture Trade project, which intends to incorporate several disciplines and sustainability frameworks to evaluate all three pillars of sustainability – environmental, social and economic – in hopes of guiding consumers toward sustainable seafood.
Ecosystems are fundamental for both social and economic systems, while poverty is often the underlying factor for environmental degradation. There is therefore a complex interaction of bottom-up effects, such as failing renewable resources (fish stocks, for example), as well as top-down effects, such as unsustainable harvesting to maintain livelihood (hunting of bush meat). Failing to acknowledge this can result in a constant chain of false solutions, exemplified by the recent biofuel hype.
Perspectives
Drastic changes will be needed to feed an additional 2.3 billion people with ever-scarcer resources up to the year 2050. The challenge will be to implement these changes in a world that currently allocates its resources toward practices such as tuna fattening and biofuel production from fish processing coproducts. To do so, we need to implement the real costs of resource depletion and environmental degradation into our valuation system or we will push our planet out of its current stability domain.
(Editor’s Note: This article was originally published in the September/October 2010 print edition of the Global Aquaculture Advocate.)
Now that you've reached the end of the article ...
… please consider supporting GSA’s mission to advance responsible seafood practices through education, advocacy and third-party assurances. The Advocate aims to document the evolution of responsible seafood practices and share the expansive knowledge of our vast network of contributors.
By becoming a Global Seafood Alliance member, you’re ensuring that all of the pre-competitive work we do through member benefits, resources and events can continue. Individual membership costs just $50 a year.
Not a GSA member? Join us.
Authors
-
Patrik Henriksson, M.S.
Leiden University
Centrum voor Milieuwetenschappen Leiden
CML/Industriele Ecologie
Van Steenis Gebouw, Einsteinweg 2
2333 C.C. Leiden, The Netherlands -
David C. Little, Ph.D.
Institute of Aquaculture
University of Stirling
Stirling, Stirlingshire, United Kingdom -
Max Troell, Ph.D.
Beijer Institute of Ecological Economics
Royal Swedish Academy of Sciences
Stockholm, Sweden -
Dr. Rene Kleijn
Leiden University
Centrum voor Milieuwetenschappen Leiden
CML/Industriele Ecologie
Van Steenis Gebouw, Einsteinweg 2
2333 C.C. Leiden, The Netherlands
Tagged With
Related Posts
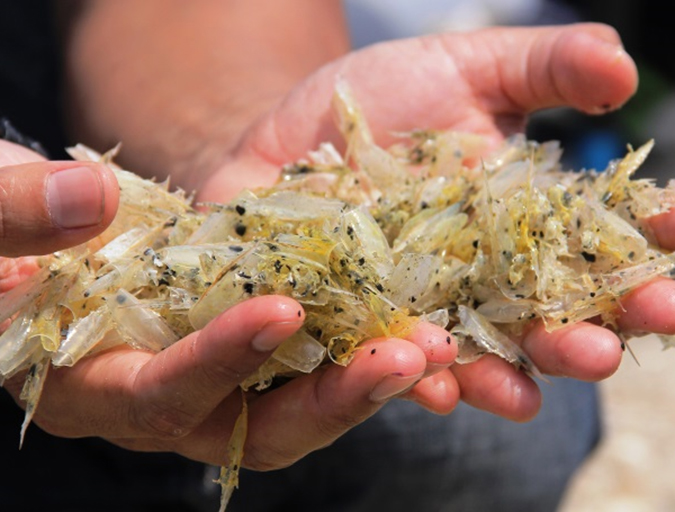
Health & Welfare
A holistic management approach to EMS
Early Mortality Syndrome has devastated farmed shrimp in Asia and Latin America. With better understanding of the pathogen and the development and improvement of novel strategies, shrimp farmers are now able to better manage the disease.
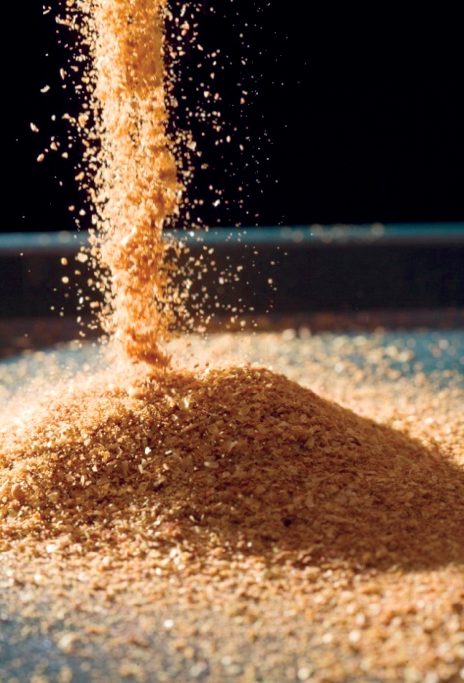
Aquafeeds
A look at corn distillers dried grains with solubles
Corn distillers dried grains with solubles are an economical source of energy, protein and digestible phosphorus to reduce feed costs and fishmeal usage.
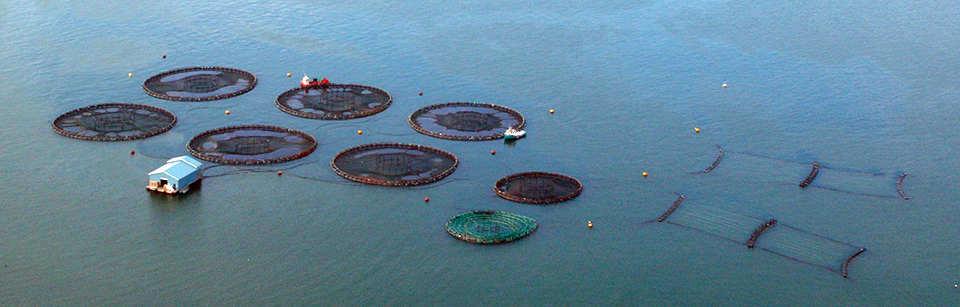
Responsibility
A look at integrated multi-trophic aquaculture
In integrated multi-trophic aquaculture, farmers combine the cultivation of fed species such as finfish or shrimp with extractive seaweeds, aquatic plants and shellfish and other invertebrates that recapture organic and inorganic particulate nutrients for their growth.
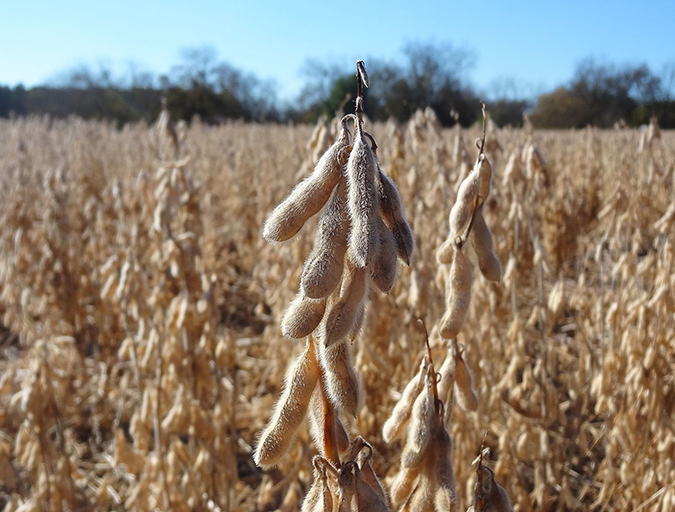
Aquafeeds
A look at phospholipids in aquafeeds
Phospholipids are the major constituents of cell membranes and are vital to the normal function of every cell and organ. The inclusion of phospholipids in aquafeeds ensures increased growth, better survival and stress resistance, and prevention of skeletal deformities of larval and juvenile stages of fish and shellfish species.