Shallow culture resulted in strong macroalgae growth rates
In shrimp farming, only 25 to 30 percent of the nitrogen and phosphorus from formulated feeds and fertilizers are incorporated by the shrimp, and most is leached and lost in the culture water. Selecting species for culture that can reuse the nutrients and maximize production is essential for more sustainable systems.
The cultivation of macroalgae associated with other aquatic organisms has gained momentum due to the increasing focus on sustainable systems with nutrient recycling. As inorganic consumers, macroalgae use nitrogen and phosphate compounds in the water for their growth. Several studies have shown a better performance of macroalgae when cultivated in shrimp farm effluent and/or in integrated systems.
This article – summarized from the original publication (Carvalho, A. et al. 2023. Growth of the Macroalgae Ulva lactuca Cultivated at Different Depths in a Biofloc Integrated System with Shrimp and Fish. Phycology 2023, 3(2), 280-293) – presents the results of a study to evaluate the cultivation of the green macroalgae Ulva lactuca in different water depths in a biofloc-integrated system with shrimp and fish, and to determine how macroalgae influence total suspended solids.
Study setup
The objective of this work was to evaluate different depths of culture structure for the macroalgae U. lactuca in an integrated system with Pacific white shrimp (Litopenaeus vannamei) and Nile tilapia (Oreochromis niloticus) in a biofloc system. The study was conducted in a greenhouse at the Marine Aquaculture Station (Estação Marinha de Aquicultura–EMA), Institute of Oceanography, Federal University of Rio Grande (FURG), Rio Grande, Rio Grande do Sul, Brazil.
The 70-day experiment involved six culture systems each with a 16-cubic-meter shrimp tank, a 3-cubic-meter fish tank and a 3-cubic-meter algae tank, with water recirculation between all the tanks. Two algae culture treatments were used, including a shallow float (10 cm depth) system and a bottom float system at 30 cm from the water surface. For detailed information on the study setup, refer to the original publication.
Results and discussion
The biomass of macroalgae in the shallow treatment showed, throughout the experimental period, a significant difference (p ≤ 0.05) from the bottom treatment. The bottom float treatment showed a decreasing trend in macroalgae biomass throughout the experimental period (Fig. 2).
In the 70 days of the experiment, the relative growth rate of macroalgae in the shallow float treatment was 0.14 ± 0.14 percent per day, with an increase in biomass in the first weeks of culture (up to 0.95 ± 0.54 percent per day) and a decrease in biomass between sampling on days 41 and 55 of culture. At the end of the experiment, the shallow treatment showed a gain in macroalgae biomass. There was no difference in the performance of fish and shrimp between the treatments during the 70 days of culture.
Integrated culture requires that water quality conditions meet the optimal levels for the cultivation of all species produced so that stress and poor development do not occur. The use of a mature biofloc inoculum with the establishment of bacteria and the presence of nitrate in this experiment provided high concentrations of total ammoniacal nitrogen and nitrite, which were controlled during culture, and no water quality problems were observed. As there was no control treatment without macroalgae, the uptake of nutrients by macroalgae was not verified. However, since there was no difference in the nitrogen content between the treatments, the loss of biomass in the bottom treatment did not cause nitrogen problems. The biomass of macroalgae used in the experiment was also low compared to the entire volume of the system, probably not causing significant nutrient uptake.
In this study, solids were deposited on the macroalgae and were not accounted for in the water quality analysis, with an overall average increase of 39.4 percent and 40.1 percent in total suspended solids in the shallow and bottom float treatments, respectively. Even when the total suspended solids concentrations were higher than 300 mg per liter and clarifiers were used, the solids were not removed from the system. The water going to the clarifier contained only the solids in suspension in the water column. The formation of this physical barrier of macroalgae may serve as a substrate for bacteria and help support and improve water quality, but little is known about this relationship or the chemical effects between macroalgae and solids.
In addition to the deposition, the production of solids from feces, feed residues, and growth of bacterial biomass was continuous throughout the days of culture. High concentrations of solids were found on days 40 to 48, exceeding 400 mg per liter total suspended solids, requiring the permanent use of clarifiers. The accumulation of solids in this period may have influenced the decrease in biomass of the macroalgae between sampling on days 41 and 55. The high organic load may prevent light from entering the water, decreasing the macroalgae’s photosynthetic efficiency and overall performance. Our results suggested that better U. lactuca performance integrated into a BFT system can be obtained from waters with TSS concentrations lower than 300 mg per liter by using clarifiers.
The increase in depth of the bottom float structures (15 to 25 cm) provides more space for macroalgae movement and greater carrying capacity for the macroalgae biomass. However, according to Luo et al., the biofloc system has great light limitations, with ammonia removal occurring predominantly by bacteria, as they do not need much light and can develop better in the system. Reis et al. studied with different colors and wavelengths for the culture of L. vannamei shrimp and evaluated the penetration of each wavelength at the surface and at 20 and 40 cm of depth.
These authors showed that the light penetration decreased with depth due to reflection or absorption by suspended particles in the water. Wavelengths of 79.05 ± 42.00 µmol/m2/m and of 20.45 ± 23.40 µmol/m2/m are absorbed, respectively, at the surface and at 20 cm depth, in white light. This decrease of light in the water column may be a determinant in reducing macroalgae growth, causing the loss of biomass seen in the bottom float treatment.
Despite the deposition of solids on the macroalgae in both treatments, the shallow float treatment (5 to 10 cm) provided better conditions for macroalgae growth. The proximity to the surface probably allowed the macroalgae to capture more light for photosynthesis. The proximity to the surface and the adaptation of the macroalgae to the biofloc environment before the beginning of the experiment were possibly determining factors for better performance.
Better management and water quality parameters still need to be established for maximum macroalgae growth to occur in integrated biofloc culture. The use of clarifiers to remove solids and the maintenance of a concentration of 100 mg per liter of total suspended solids would probably favor greater light input. The shallow float allowed the macroalgae to grow in culture, and the use of aeration within the macroalgae structure would probably be more efficient in moving the macroalgae in the structure, and less settling of solids would occur. An improved management protocol could include partial harvests, which can decrease the density, promoting greater light penetration and nutrient availability.
In our study, tilapia were added to both treatments to consume the solids, but there was no quantification of the solids consumption. However, the addition of an organic consumer along with the macroalgae may help to reduce the solids and improve the light incidence in the water, favoring the growth of the macroalgae.
Perspectives
The integrated culture in a biofloc system presents distinct characteristics compared to conventional cultures in clear water due to the high load of solids and nutrients. The insertion of the macroalgae in the integrated biofloc system showed deposition of solids on the macroalgae, decreasing the concentrations of total suspended solids and avoiding solids exiting the system by clarification.
Even with this result, there was growth of the macroalgae U. lactuca in an integrated system with the shrimp L. vannamei and Nile tilapia O. niloticus, showing the algae culture viability at depths of up to 10 cm in a biofloc system with an average TSS concentration of 300 mg per liter.
Now that you've reached the end of the article ...
… please consider supporting GSA’s mission to advance responsible seafood practices through education, advocacy and third-party assurances. The Advocate aims to document the evolution of responsible seafood practices and share the expansive knowledge of our vast network of contributors.
By becoming a Global Seafood Alliance member, you’re ensuring that all of the pre-competitive work we do through member benefits, resources and events can continue. Individual membership costs just $50 a year.
Not a GSA member? Join us.
Author
-
Dr. Andrezza Carvalho
Corresponding author
Marine Aquaculture Station, Institute of Oceanography, Federal University of Rio Grande–FURG, Rio Grande 96210030, RS, Brazil[109,111,99,46,108,105,97,109,116,111,104,64,115,97,103,97,104,99,97,122,122,101,114,100,110,97]
Tagged With
Related Posts
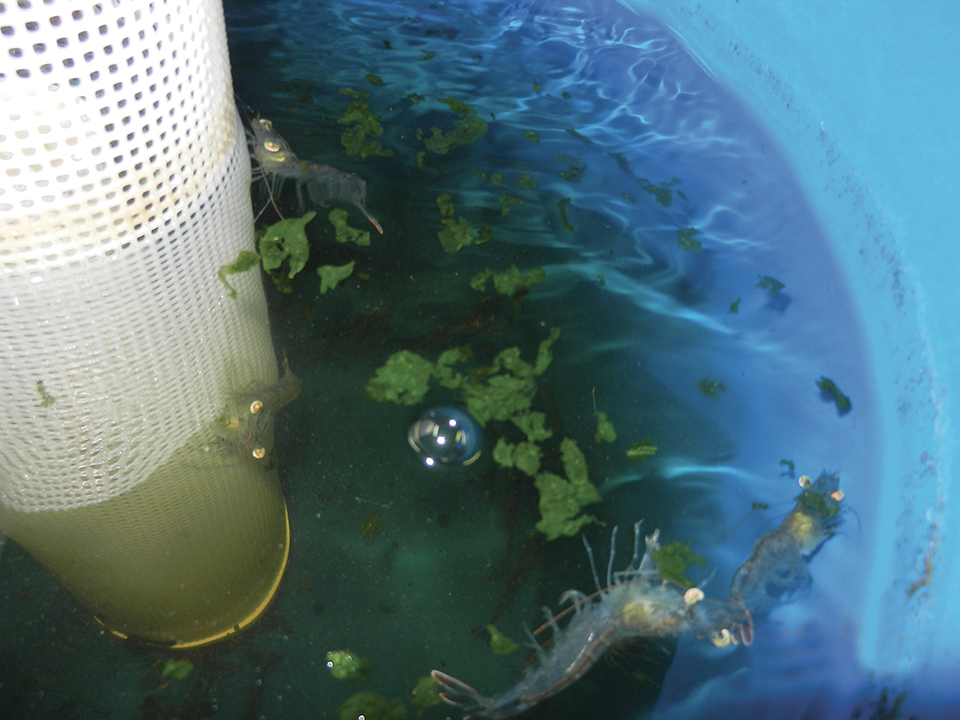
Aquafeeds
Utilization of Ulva lactuca studied in white shrimp diets
Can fresh U. lactuca harvested weekly from a land-based integrated multi-trophic aquaculture system be utilized as a nutritional supplement or partial replacement for pelleted feeds in the production of Litopenaeus vannamei?
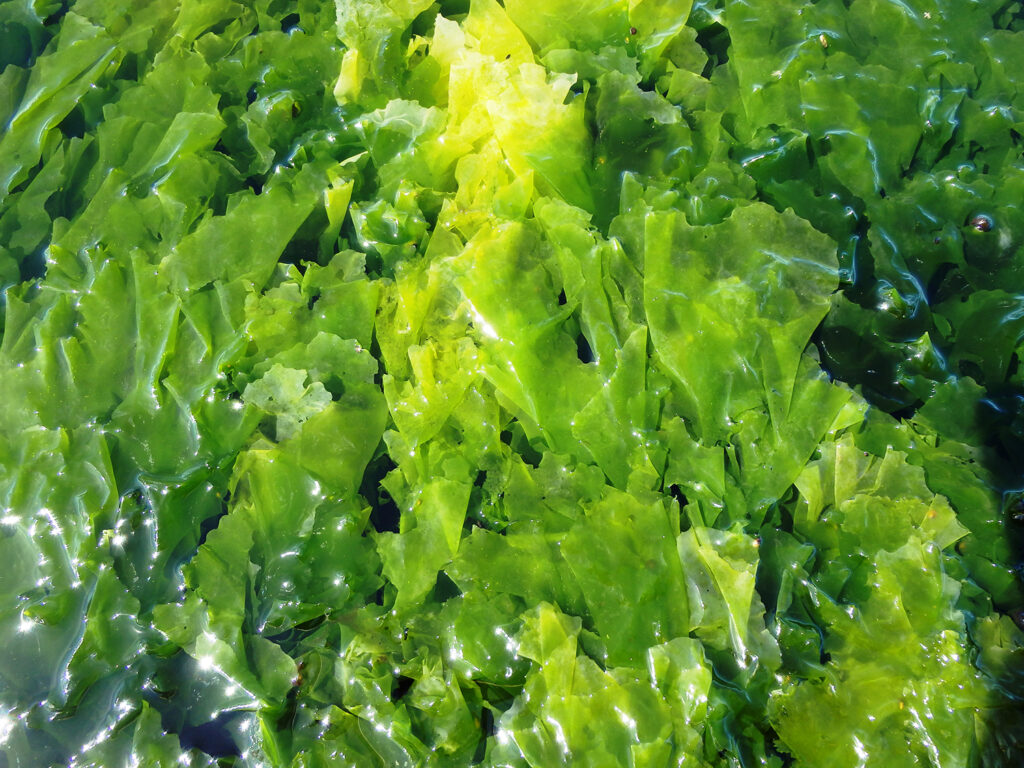
Responsibility
From smolt wastewater to algae production: an economic approach on aquaponic production
In the next article from the GAIN project, producing algae from smolt wastewater can increase salmon farms’ profits and maximize nutrient use.
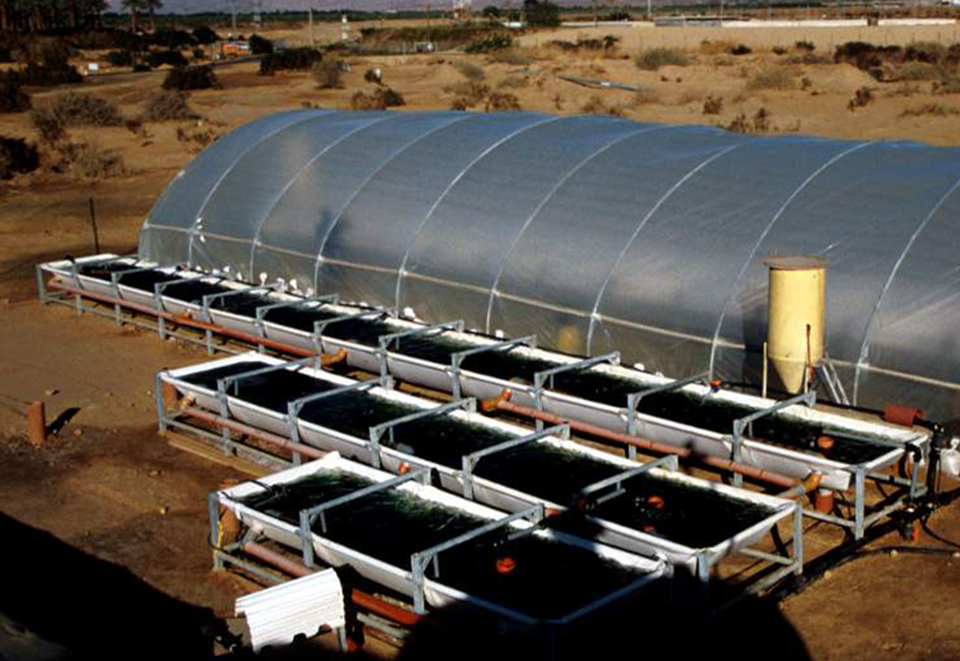
Responsibility
Integrated fish-seaweed culture systems
The use of integrated mariculture systems incorporating seaweed biofilters addresses the impact of discharges, promoting more sustainable production.
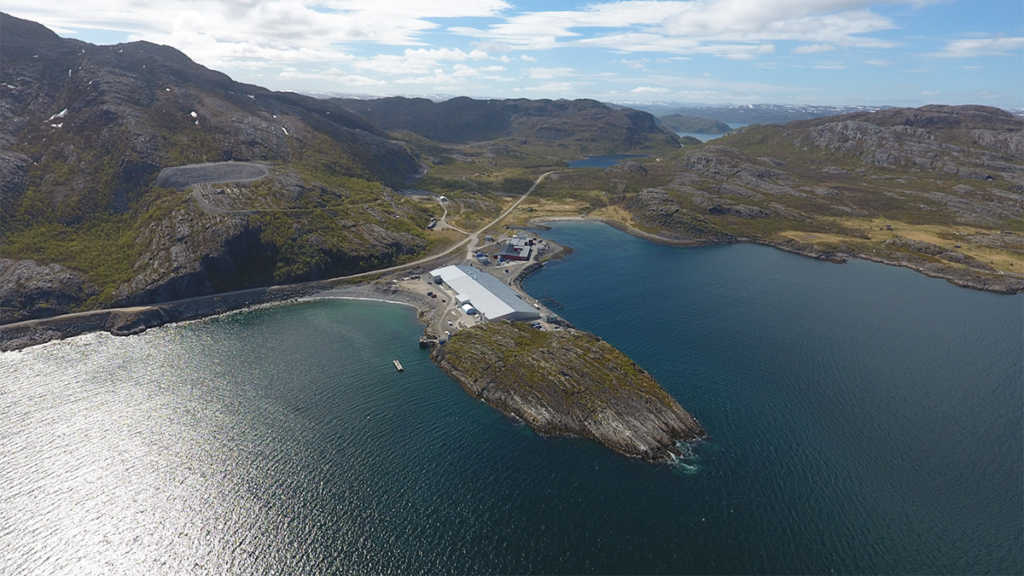
Responsibility
Sludge report: Finding value in Norway’s aquaculture waste
Bioretur converts aquaculture waste, or sludge, into fertilizer as demand for “circular economy” technologies grows.