Major challenge will be improving multiple traits in aquatic species
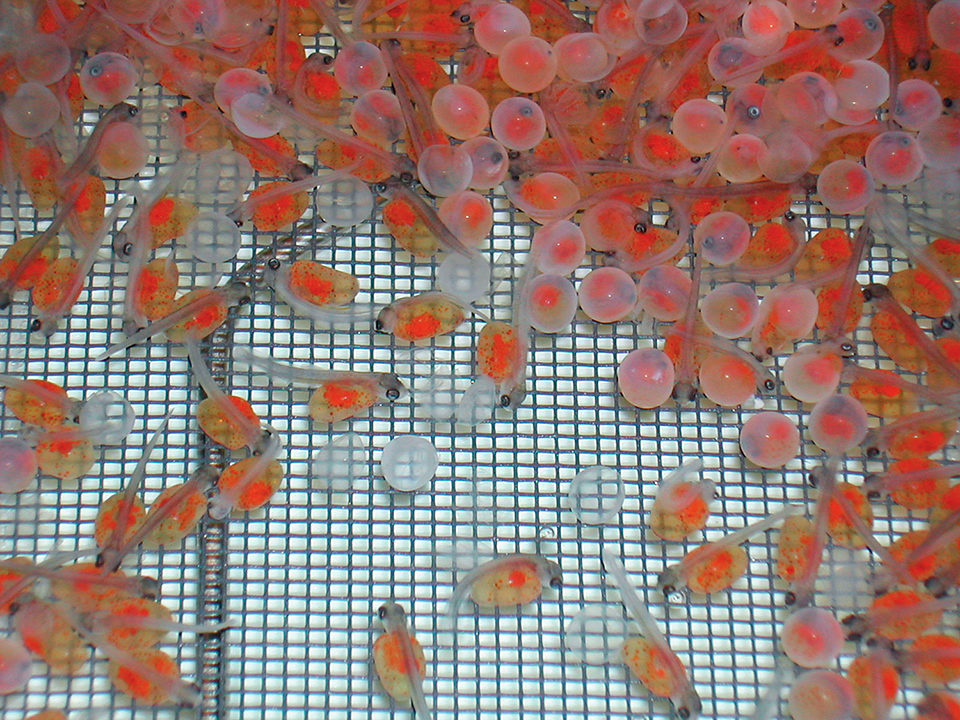
In the transition from wild harvest of a product to agricultural production, genetic improvement is always an important developmental step. The first stage is usually the process of domestication. Further genetic improvement in agriculture or aquaculture typically has a human focus on enhanced production characteristics.
Just as in terrestrial production scenarios, aquaculturists value faster growth, improved efficiency, less loss to disease, and high quality. Several tools are available to accomplish such genetic improvements.
Domestication
Most stocks of the aquatic organisms most widely grown in captivity are domesticated to some degree, and a few have undergone further genetic improvement. Just as terrestrial livestock and crops are bred to be not only productive, but productive in convenient proximity to humans, aquatic plants and animals are now undergoing these same pressures.
Although the domestication process is arguably at a much earlier stage for aquatic organisms than their terrestrial counterparts, aquaculture is undergoing the process at a time when the technologies and tools available could remarkably speed up domestication and genetic improvement.

Selective breeding
The basic tool available for genetic improvement, selective breeding, entails choosing the animals with the highest genetic value as breeders for the next generation. To determine this genetic value, populations are raised in a standardized environment so identified differences will be due to differences in genes, not the environment.
This sort of testing is expensive in terms of the facilities required to standardize the environment, maintain consistent rearing, and measure hundreds to thousands of groups. The elite performers are selected for breeding to produce subsequent generations.
This “classical quantitative genetics” approach has been around since the early 20th century, and most of the genetic gains realized through today can be attributed to traditional selective-breeding methods. Yet to think this branch of science has remained static would miss the mark greatly. Improvements in experimental designs, tagging technologies to identify individuals and groups, and computational tools have revolutionized the accuracy and power of these classical techniques.
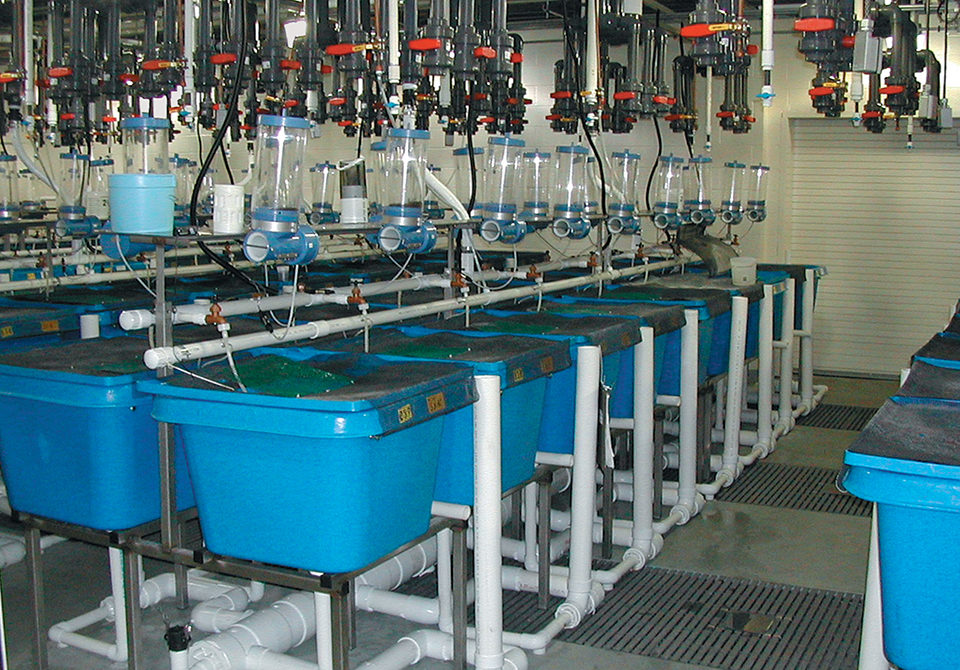
Genetic markers
Genetic markers include several classes. Amplification fragment length polymorphisms and microsatellite markers are two of the most frequently used genetic markers in aquaculture. They reflect regions of DNA that are used to keep track of specific locations within the genome. The markers are distributed throughout the DNA of an organism and transmitted from parent to offspring.
Genetic markers are useful for determining parentage because offspring share markers with their parents. They are also expected to be useful for identifying regions of DNA that confer performance advantages.
If a particular marker is always found associated with resistance to a coldwater bacterial disease, for example, selection for breeding could be based on the presence of this marker without doing a challenge trial under tightly controlled environmental conditions. This application of selective breeding is referred to as marker-assisted selection.
An important consideration for marker-assisted selection is that traditional approaches relying on direct trait measurements in organisms are expensive. Furthermore, elite performers in disease challenges are usually not used for breeding for fear of transmitting disease, but their close relatives could be used.
A similar situation occurs if traits of interest are measured after test animals are slaughtered. Individuals with high meat quality or fillet yield may not be available for breeding after the traits are measured.
Genetic engineering
Genetic engineering is a broad heading that includes chromosome set manipulations and transgenesis. Chromosome set manipulation is a group of techniques in which sets of chromosomes in organisms are modified.
Whereas normal organisms have two sets of chromosomes – one from each parent – one type of chromosome set manipulation can result in triploids, organisms with three sets of chromosomes. These animals are generally sterile, which can be advantageous under conditions where reproduction is undesirable.
This technology has been applied in the United States to the nonindigenous weed-controlling grass carp to prevent unwanted reproduction. Chromosome manipulation has also been used in oysters, which typically undergo a loss of quality during the spawning season.
Transgenesis
Transgenesis refers to the technique of incorporating a gene or genes through biotechnological methods, not breeding. Soybean plants that have been made resistant to the effects of herbicides are an example of transgenic crops. With a gene from bacteria transferred into and expressed in the plants, their use reduces labor costs for cultivating and weeding. Golden rice, a transgenic rice plant, has three foreign genes inserted from bacteria and daffodils to allow the production of vitamin A in the rice.
The Glofish, a fluorescent zebra-fish which came on the U.S. market in January 2004, is the first transgenic aquatic organism to be marketed. The proteins responsible for making the zebrafish fluoresce are transgenes derived from jellyfish and coral. These fish are not intended for food.
Atlantic salmon with a growth hormone gene from Chinook salmon and a promoter, or switch, from the ocean pout are currently being reviewed by the United States Food and Drug Administration for approval as food fish. Research on other aquatic transgenics with the potential for greater disease resistance, enhanced nutritive value, and other characteristics is ongoing around the world.
Ongoing challenges
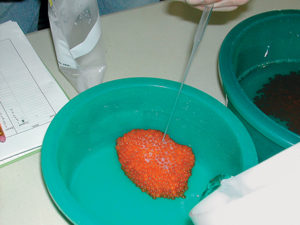
The use of classical breeding techniques remains the foundation upon which genetic improvement will be built for the foreseeable future. These techniques will continue to be refined to offer faster rates of improvement in performance. Incorporating marker information into selective breeding is a challenge, both in terms of the practicalities of identifying marker-trait associations and how to incorporate the information.
Perhaps the major ongoing challenge will be to improve multiple traits in aquatic species. Fast, efficient growth; disease resistance; and product quality are major traits of interest, but the relationships among these traits are not well known. Since the genetic values for traits are not well defined, or multiple traits of interest are not measured on the same individuals, the genetic relationships have not been determined.
In limited cases, the relationships among a subset of these traits are known. For example, the genetic relationship between growth and resistance to viral hemorrhagic septicemia (VHS) in one farmed population of rainbow trout, Oncorhynchus mykiss, was shown to be negative. This unfortunately suggested that simply selecting for improvement in growth would result in lowered resistance to VHS.
Especially in cases like this, knowledge of markers associated with disease resistance and improved estimates of genetic value for multiple traits will enable solutions. Current developments in genetic improvement hold the promise that the relatively rare individuals with superior performance for several traits of interest can be identified and selected for breeding.
(Editor’s Note: This article was originally published in the February 2005 print edition of the Global Aquaculture Advocate.)
Now that you've reached the end of the article ...
… please consider supporting GSA’s mission to advance responsible seafood practices through education, advocacy and third-party assurances. The Advocate aims to document the evolution of responsible seafood practices and share the expansive knowledge of our vast network of contributors.
By becoming a Global Seafood Alliance member, you’re ensuring that all of the pre-competitive work we do through member benefits, resources and events can continue. Individual membership costs just $50 a year.
Not a GSA member? Join us.
Author
-
Jeffrey T. Silverstein, Ph.D.
USDA/ARS National Center for Cool and Cold Water Aquaculture
11861 Leetown Road
Kearneysville, West Virginia 25430 USA[118,111,103,46,97,100,115,117,46,115,114,97,46,97,119,99,99,99,110,64,115,114,101,118,108,105,115,106]
Related Posts
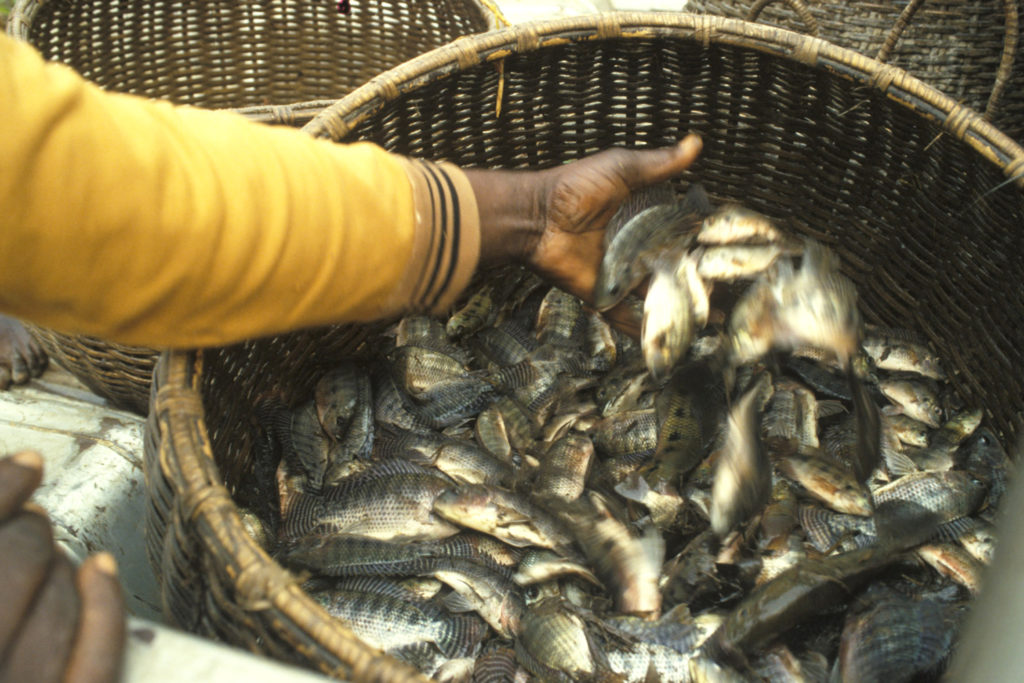
Responsibility
A helping hand to lend: UK aquaculture seeks to broaden its horizons
Aquaculture is an essential contributor to the world food security challenge, and every stakeholder has a role to play in the sector’s evolution, delegates were told at the recent Aquaculture’s Global Outlook: Embracing Internationality seminar in Edinburgh, Scotland.
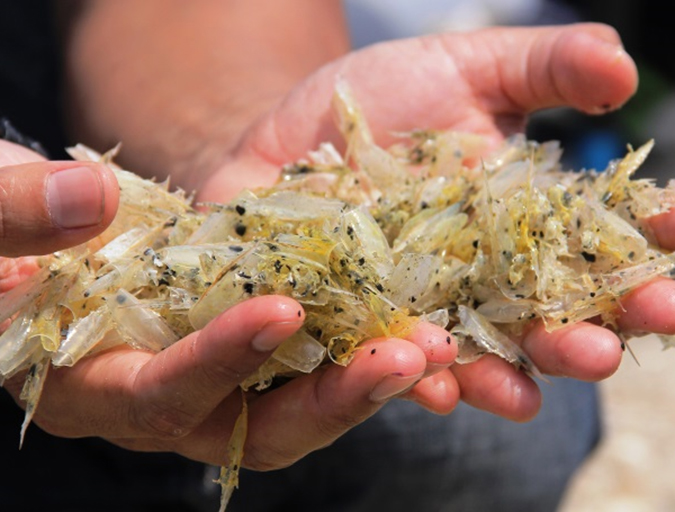
Health & Welfare
A holistic management approach to EMS
Early Mortality Syndrome has devastated farmed shrimp in Asia and Latin America. With better understanding of the pathogen and the development and improvement of novel strategies, shrimp farmers are now able to better manage the disease.
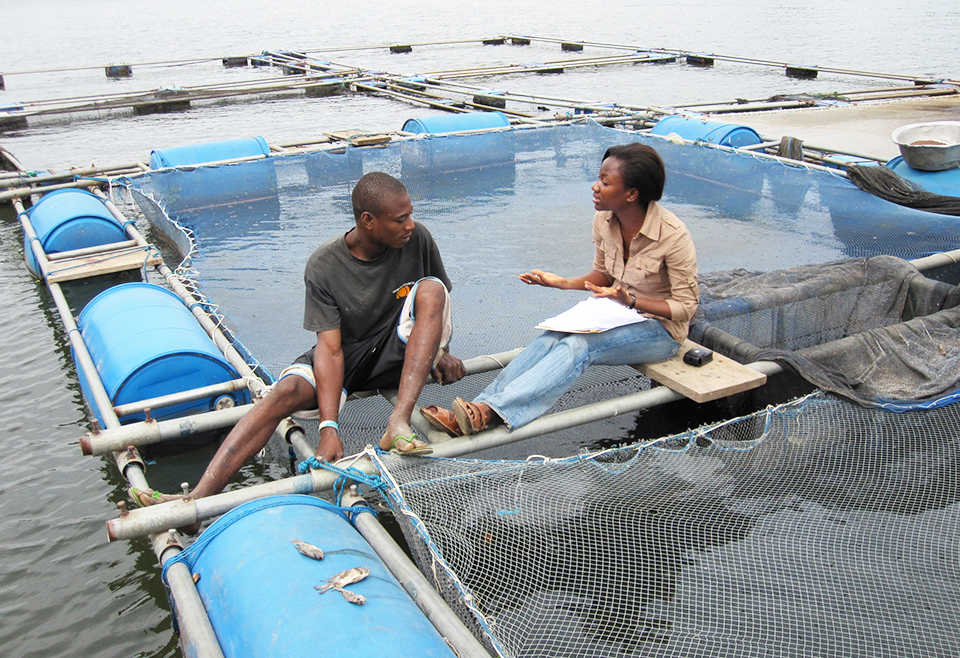
Health & Welfare
A look at tilapia aquaculture in Ghana
Aquaculture in Ghana has overcome its historic fits and starts and is helping to narrow the gap between domestic seafood production and consumption. Production is based on Nile tilapia.
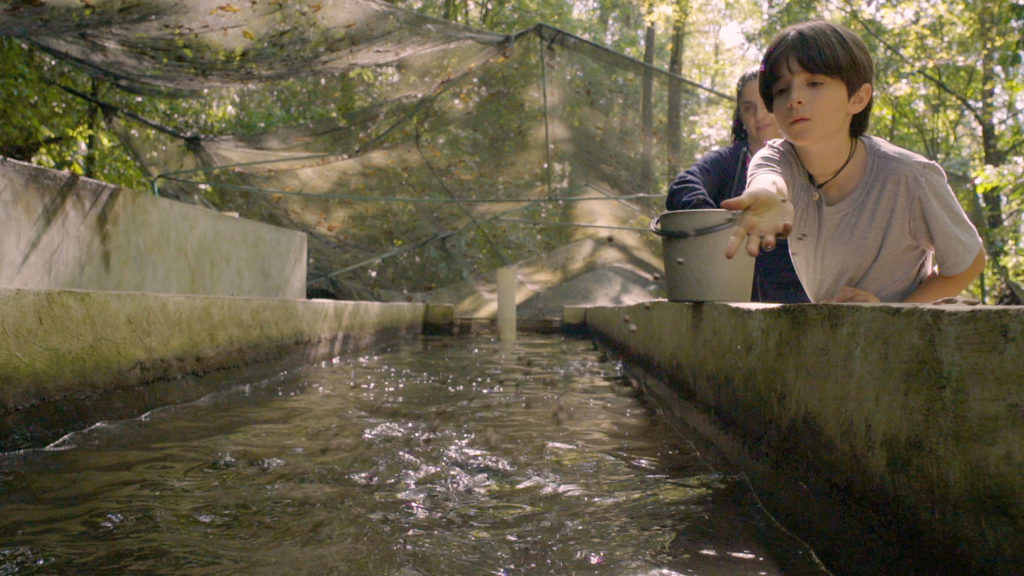
Intelligence
A motive, and a market, for farmed fish in Mexico
Boasting ample areas for aquaculture and a robust domestic demand for seafood – not to mention its close proximity to the U.S. market – a land of opportunity lies in Mexico. Fish farming is primed to meet its potential south of the border.