Solar panels, reservoirs maintain water temperatures in experimental RAS System
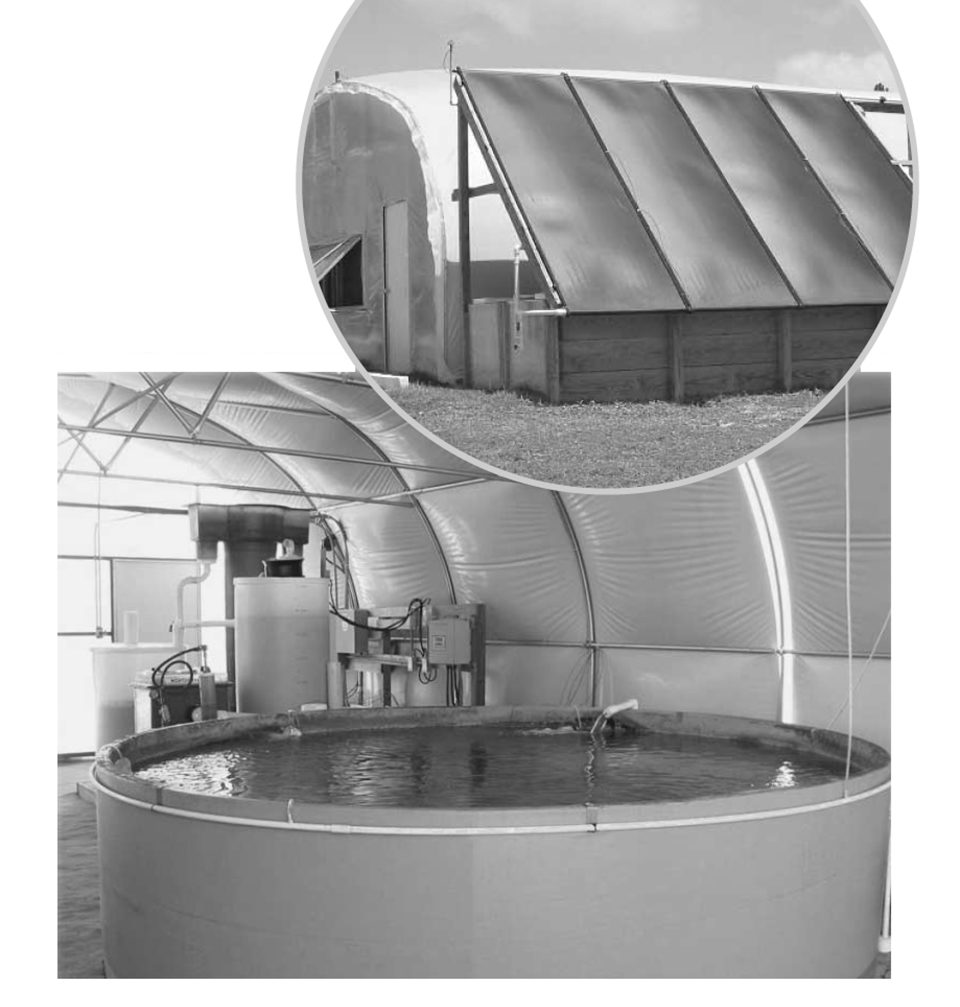
Florida State University researchers, in collaboration with the United States Department of Agriculture Agricultural Research Service and Harbor Branch Oceanographic Institution, are evaluating whether off-the-shelf flat-plate solar collectors can maintain stable recirculating aquaculture system (RAS) temperatures and if it is cost-effective to do so. Well-designed recirculation systems could be ideal for maintaining elevated production temperatures, due to far less heat loss than flow-through or outdoor systems.
Energy costs
After feed, energy is the second-largest variable cost of recirculation production. The application of a blend of conventional and alternate energy technologies to reduce this cost should directly result in increased profits. Dr. Ron Malone of Louisiana State University in Louisiana, USA, has touted air-lift pumping over conventional pumping for years as a way to reduce energy costs.
If solar water heating can effectively compete with resistive heating in thermal performance, then the question becomes one of higher capital costs in solar systems versus higher operating costs in conventional systems. Florida State University (FSU) determined to first compare conventional resistive heating with flat-panel solar heat collectors and insulated reservoirs for maintaining optimal production temperatures (OPT).
Optimal production temperatures
Why heat at all? Maintaining OPT allows 12 months of full production per year, predictable harvest dates, stable system operation, and efficient feed conversion.
OPT is a concept based upon the idea that cold-blooded species grow faster when kept warm. Unfortunately, the warmer water, the less oxygen it holds. At some point, metabolic oxygen demand exceeds oxygen availability and the animals struggle or perish.
OPT, which varies by species, is the relative balance point among rapid growth, efficient feed conversion, available oxygen, elevated stress and disease losses, heating energy costs, and profitability. For southern flounders, one of FSU’s target species, the OPT ranges 24 to 26 degrees-C. North Florida experiences average temperatures below 20 degrees-C from mid-November through mid-March, thus heating is necessary or growth declines dramatically.
Solar systems
Reservoir volume, surface area, insulation, exposure to weather, distance to components, and heat exchanger efficiency all affect how well and how long a solar system will function prior to requiring backup heating. Trade-offs must be made between durability, ease of repair and maintenance, cost of construction and operation, capacity, performance, and efficiency.
North Florida receives daily average solar energy of 4 kWh per square meter between November and February. Solar energy is not constant, so one must capture enough to last through the night and extended periods of cloud cover and rain. Flat-panel collectors must be pointed south ± 5 degrees and rotated off vertical to latitude +15 degrees for best effect during the winter heating cycle.
Greenhouse RAS
With U.S. Department of Agriculture Agricultural Research Service funding, the authors built two 9- x 15-meter inflated greenhouses at the Florida State University Marine Laboratory in Turkey Point, Florida, and installed a 10-kl insulated RAS package and 36-kW resistive heating system with controllers in each. To one was added 35-square-meter flat solar panels, two 10-kl reservoirs, a heat exchanger, pumps and controllers.
The solar collector surface area was designed to be twice the production tank surface area, since collection occurs only during the day and heating is ongoing day and night. Similarly, reservoirs for heat storage were also designed at double the production system volume.
A Campbell data-logging system and array of sensors throughout monitored the systems. When allowed to heat and cool at ambient temperatures, both recirculation systems performed thermally in virtual lock step, indicating both greenhouse systems were equivalent. Patterns of diurnal variations among temperature, humidity, and energy use were precise between the two production tanks.
Preliminary results
Between January and April 2003, the data loggers reported that the solar system maintained the production system at 25 ± 30 degrees-C with reservoir temperatures of 35 to 46 degrees-C, despite ambient air temperatures as low as minus-5. Between June and October, the standard greenhouse evaporative cooling system kept the tanks at 26 ± 3 degrees-C, despite the location on the humid Gulf of Mexico coast.
In August, warm night temperatures led researchers to explore using the solar collectors as radiators for six hours a night. Radiative cooling via the collectors did occur, but only down to ambient air temperature plus 3 to 5 degrees-C. It was unclear whether this was cost-effective. The authors will review this technique in detail this summer.
Although the two systems’ thermal patterns appear almost identical, resistive heating costs far more than pumping water through the solar panels and heat exchanger (Fig. 1). During a six-week trial run this past winter, resistance heating the RAS used 4,000 kWh more power than solar heating to achieve the same thermal signature. Despite temperatures that dipped below minus-5 degrees-C, the backup resistance heaters in the solar-heated RAS were only required for two days.

Cost effectiveness
The flat-panel solar heating technology appeared to match conventional heating in performance. Whether solar heating is cost-effective remains undetermined at this point. Solar heating costs more in start-up capital, but costs less to operate.
Many farmers are reluctant to impound several times as much water as they actually use for production. However, this may be more of a mental stretch than an economic problem, since liners cost so little, and reservoirs need only minimal additional water to beat evaporation.
Utilizing this year’s data set, Florida State University will begin to develop a descriptive and predictive model for solar-heating an RAS. This will allow exploration of whether money would be best spent on more insulation, more collector panels, alternate reservoir designs, etc. to further reduce energy costs while maintaining satisfactory performance. Much of the solar system can be expected to last 10 or more years. In order to evaluate realistic estimates of cost efficiency, long-term research is the only solution.
(Editor’s Note: This article was originally published in the April 2004 print edition of the Global Aquaculture Advocate.)
Now that you've reached the end of the article ...
… please consider supporting GSA’s mission to advance responsible seafood practices through education, advocacy and third-party assurances. The Advocate aims to document the evolution of responsible seafood practices and share the expansive knowledge of our vast network of contributors.
By becoming a Global Seafood Alliance member, you’re ensuring that all of the pre-competitive work we do through member benefits, resources and events can continue. Individual membership costs just $50 a year.
Not a GSA member? Join us.
Authors
-
Jeffrey Wilcox, Ph.D.
Department of Oceanography
Florida State University
0102 OSB, West Call Street
Tallahassee, Florida 32306 USA[117,100,101,46,117,115,102,46,110,97,101,99,111,64,120,111,99,108,105,119]
-
Nancy Marcus, Ph.D.
Department of Oceanography
Florida State University
0102 OSB, West Call Street
Tallahassee, Florida 32306 USA -
George Buzyna, Ph.D.
Department of Mechanical Engineering
Florida State University
Tallahassee, Florida, USA
Tagged With
Related Posts
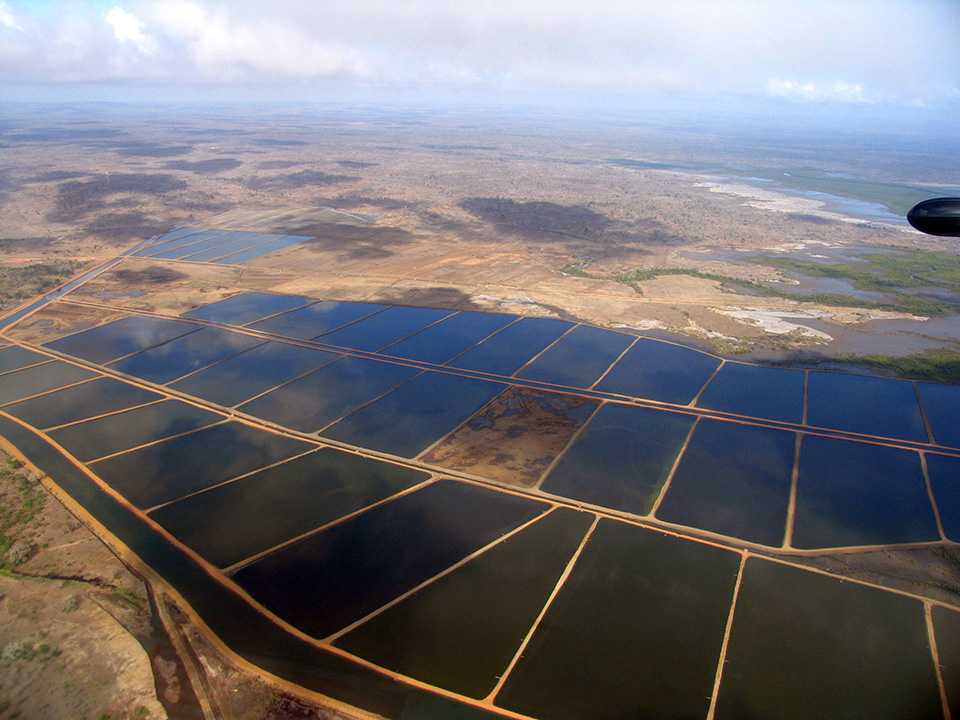
Responsibility
Aquaculture ponds hold carbon
Although 16.6 million metric tons of carbon are annually buried in aquaculture ponds, estimated carbon emissions for culture species have approached several metric tons of carbon per metric ton of aquaculture product.
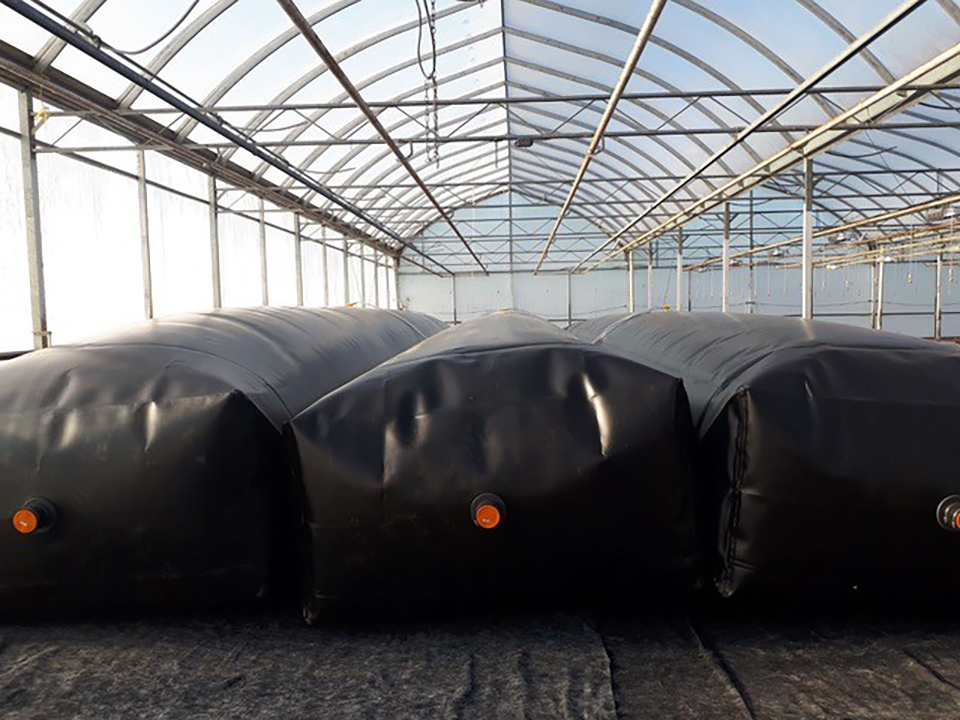
Responsibility
Canada invests in ‘clean-tech’ aquaculture
A handful of aquaculture companies in British Columbia are upgrading to "clean-tech" equipment to make their businesses more sustainable and efficient.
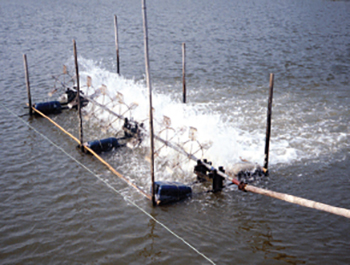
Responsibility
Efficiency of mechanical aeration
Although relatively expensive, mechanical aeration increases production. Asian-style paddlewheel aerators are widely used but are inefficient. Testing has led to more efficient designs that are now widely used in U.S. catfish and shrimp ponds.
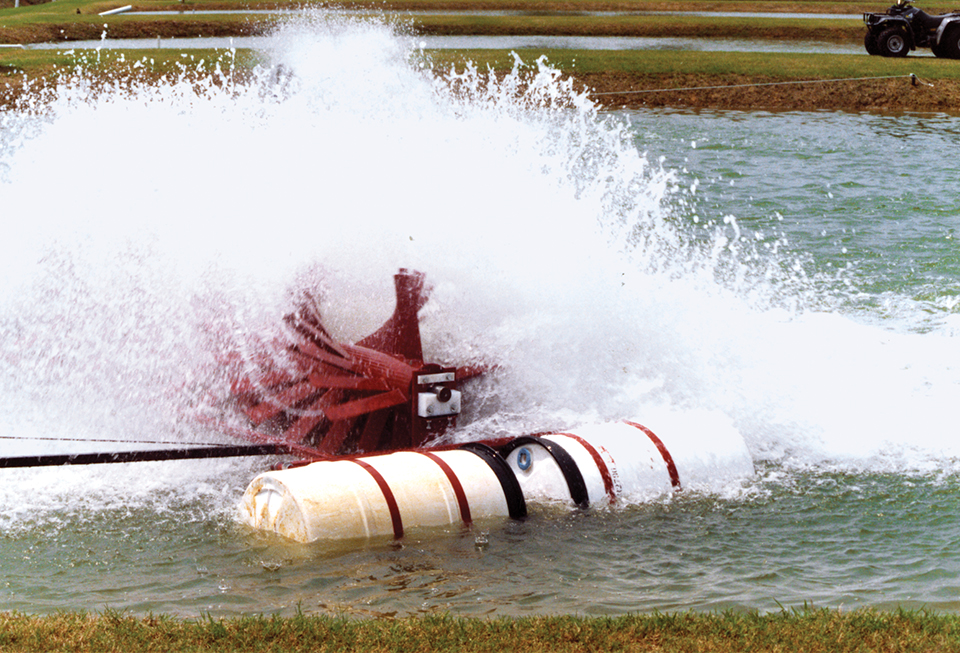
Responsibility
Direct energy use in channel catfish production
The direct energy input values for aquaculture include energy used in constructing facilities, producing seedstock and feed, powering mechanical aerators, applying feed and harvesting and processing culture animals.