Results demonstrate the efficacy of culturing shrimp in high-density biofloc systems across a range of feed inputs
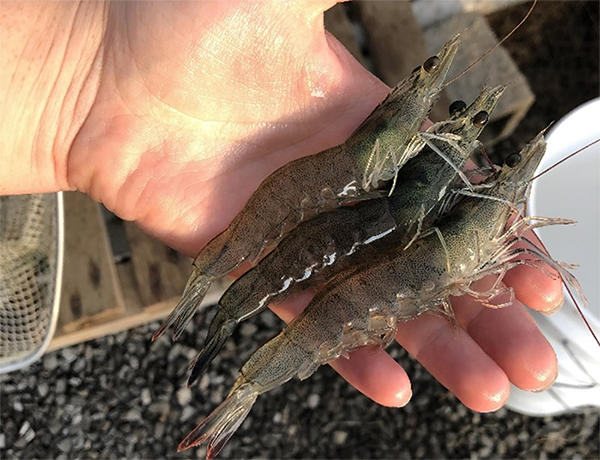
The global shrimp farming industry has expanded significantly, and production has increased to meet growing demand for affordable, high-quality protein. With this expansion, intensive production systems have become popular. And with the exception of clearwater recirculating aquaculture systems (RAS), there are typically considerable levels of natural foods in shrimp culture systems.
Biofloc technology (BFT) systems are quite popular, originally gaining recognition because of their use as a water-quality management tool. Though biofloc composition varies considerably based on light availability, carbon sourcing and microorganism population, BFT systems are generally described as a system with heterotrophic bacteria, algae, detritus and other microorganisms present.
While it is already known that shrimp are omnivorous and can consume algae and other organisms present in the culture system, this contribution has not been quantified or accurately factored into most feeding protocols. In a recent study, we demonstrated the contribution of algae and other biological aggregates (often referred to as “natural productivity”) present in shrimp biofloc rearing systems to shrimp growth. More information about this research is available in the publication titled “Feed management of Litopenaeus vannamei in a high density biofloc system.”
We designed and conducted a feeding experiment to allow us to evaluate the approximate contribution of natural productivity to shrimp growth. Our objective was to evaluate shrimp growth performance at various feed input levels in order to quantify natural productivity contribution to growth, as well as determine the most efficient feeding level. Maximum feeding levels in this experiment led to maximum growth, but these feeding levels were not the most efficient; higher feeding levels showed diminishing returns even though a growth plateau was not observed. High feed rates also increase feed wastage, which exacerbates water quality management challenges.
Study setup
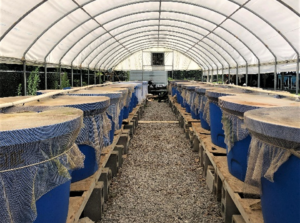
The trial was conducted in an outdoor, biofloc recirculating aquaculture system (RAS) consisting of 24, 0.75-square-meter culture tanks. Pacific white shrimp (Litopenaeus vannamei) juveniles with an initial weight of 0.17g were stocked at a density of 150 shrimp per cubic meter in polyethylene tanks and grown for 63 days. The tanks were filled with water from a shrimp production pond. Water was only replaced to compensate for evaporation losses throughout the trial, in order to create a biofloc-type experimental system.
Treatments consisted of seven varying feeding rates (30, 60, 90, 105, 120, 135 and 150 percent of body weight) which was offered four times per day (Table 1). An eighth treatment was fed the highest feeding level (150 percent) six times per day rather than the standard four (indicated by an * in the table). This eighth treatment was used because previous pond research has indicated that high feed volumes broken into more feedings per day leads to more efficient feed conversion as compared to the same feed amounts in fewer feedings per day.
Weldon, Natural productivity, Table 1
Percentage (%) of standard ration | 30 | 60 | 90 | 105 | 120 | 135 | 150 | 150* |
---|---|---|---|---|---|---|---|---|
Weekly feed (g) | 56 | 112 | 169 | 197 | 225 | 253 | 281 | 281 |
For additional information on the study, refer to the original article or contact the corresponding author.
Results and discussion
At the conclusion of the trial, higher amounts of feed and more feedings per day were shown to result in significantly larger shrimp than the lower feeding amounts, while still achieving an acceptable feed conversion ratio of 1.19. Regression analysis was performed on the final body weight of shrimp from the four lowest feeding rates to approximate what shrimp growth would have been if shrimp were given zero feed. This relationship is modeled in Fig. 1.
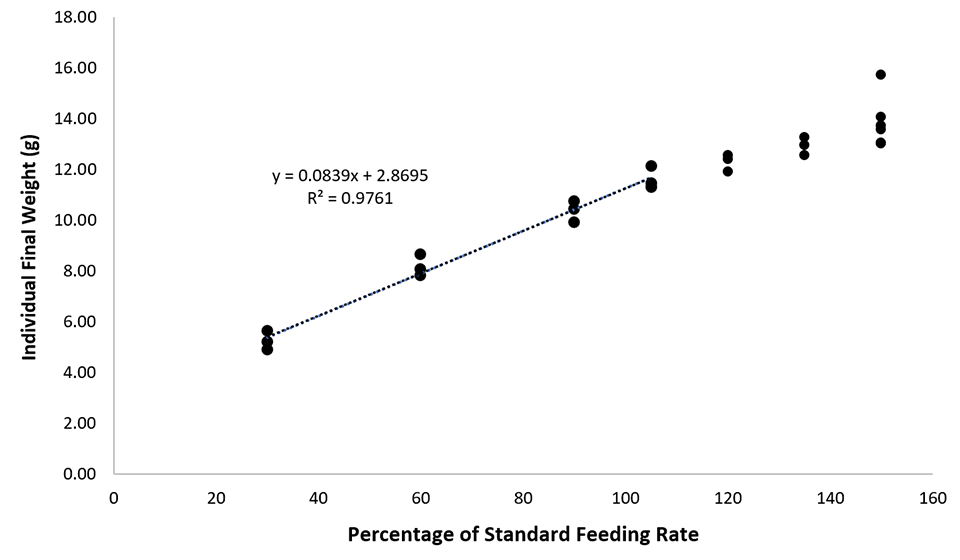
Regression analysis indicated that had shrimp not been fed, they would have weighed 2.8 grams, meaning that 2.8 grams of individual shrimp growth could be attributed to natural productivity across all treatments. This allowed us to adjust shrimp final weight to account for natural productivity when calculating protein retention from the feed with or without the estimated contribution.
Before adjusting the numbers, the data indicated that shrimp offered less feed had considerably higher protein retention percentages. However, after adjusting the numbers to remove natural productivity contribution, it was apparent that all shrimp retained the same percentage of protein from the feed.
This is important to note, because looking at raw retention numbers would imply that feeding shrimp less leads to more efficient protein retention from feed. However, that assumption would be inaccurate, as shrimp offered less feed still retained the same percentage of protein while consuming proportionately higher amounts of natural foods in relation to their total body weight. Shrimp offered more feed did not need to forage as much as the shrimp offered less, as they had ample feed available. Regardless of amount of feed offered, shrimp retained between 30 to 40 percent of protein from the feed.
As mentioned previously, shrimp are omnivores and can consume organisms commonly found in floc. This is important to consider when considering feeding strategies, as the added feed is not the only feed source in these systems. Overfeeding, even by a small amount, contributes to poor water quality and wasted resources. As Fig. 2 shows, when feed is reduced, feed conversion is more efficient as the shrimp work to capture more natural productivity, but underfeeding will lead to slow growth and smaller shrimp at harvest. Aside from the obvious advantage of saving money by avoiding feed wastage, feeding optimal amounts also avoids detrimental water quality conditions which can poorly influence shrimp growth.
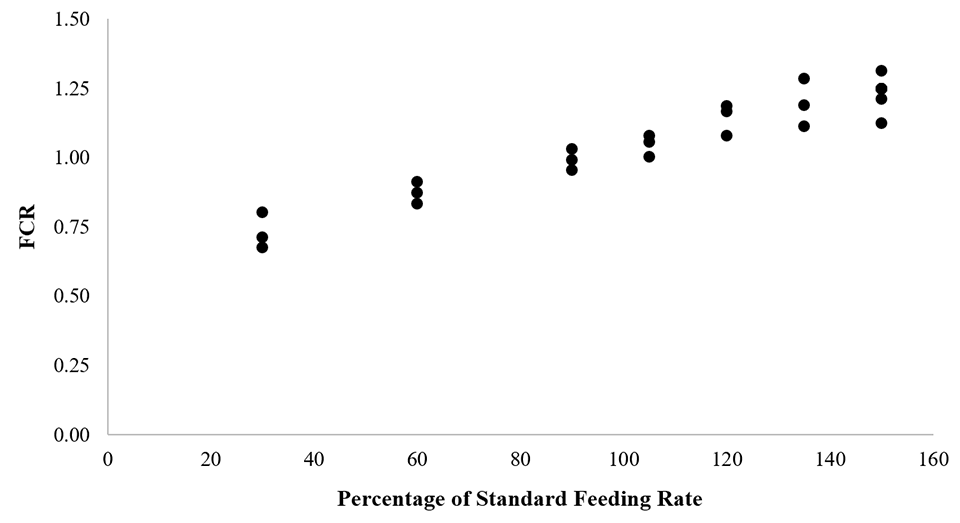
Perspectives
When natural foods are factored into feeding amounts, shrimp producers can feed an optimal amount of feed to reach market-sized shrimp in the shortest period of time while also avoiding poor water quality conditions. In our experiment, feeding rate was directly linked to final weight, with maximum feeding leading to maximum gain. However, the maximum feeding amount was not the most efficient and could contribute to poor water quality conditions in a production pond trial.
Broken line analysis was applied to shrimp final weight in order to determine where diminishing returns started. The results of this analysis showed that diminishing returns started at 101 percent of the standard feeding rate, meaning that 101 percent is the most efficient feeding rate, and feeding over that amount will lead to larger shrimp at the expense of wasted feed.
We did not raise the feeding rate high enough in this trial in order to observe a true growth plateau, as higher feeding amounts always resulted in larger shrimp. We speculate that the highest feeding rate was nearing the growth plateau, but additional research would be needed to confirm this prediction.
Because biofloc composition varies so much between culture systems and is hard to replicate without using a “seed” water sample from an existing system, we did not evaluate the composition of our floc material. Shrimp producers typically do not evaluate the floc composition of their water either, so our results can be used as a general approximation to factor into feeding rates for green water biofloc systems.
Now that you've reached the end of the article ...
… please consider supporting GSA’s mission to advance responsible seafood practices through education, advocacy and third-party assurances. The Advocate aims to document the evolution of responsible seafood practices and share the expansive knowledge of our vast network of contributors.
By becoming a Global Seafood Alliance member, you’re ensuring that all of the pre-competitive work we do through member benefits, resources and events can continue. Individual membership costs just $50 a year.
Not a GSA member? Join us.
Authors
-
Alexis Weldon, M.Sc.
Corresponding author
School of Fisheries, Aquaculture and Aquatic Sciences
Auburn University
Auburn, Alabama USA[117,100,101,46,110,114,117,98,117,97,64,54,57,48,48,119,106,97]
-
Melanie A. Rhodes
School of Fisheries, Aquaculture and Aquatic Sciences
Auburn University
Auburn, Alabama USA -
D. Allen Davis Ph.D.
Professor
School of Fisheries, Aquaculture and Aquatic Sciences
Auburn University
Auburn, Alabama USA
Tagged With
Related Posts
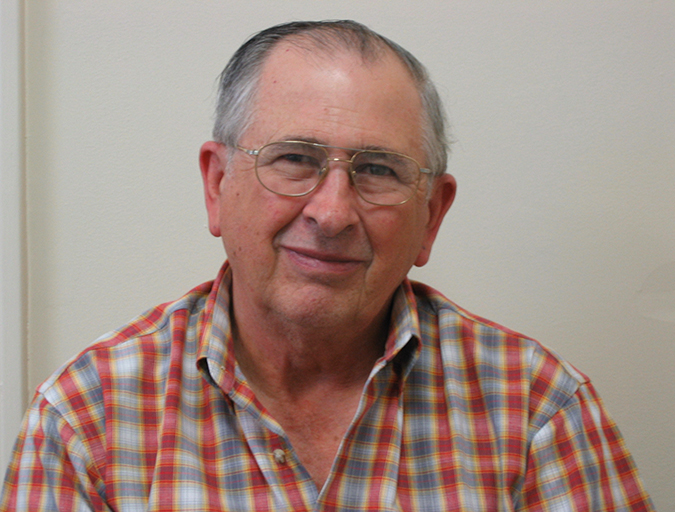
Innovation & Investment
After half a century, Auburn’s Claude Boyd to ‘retire’
Friends, colleagues and former students of longtime Auburn University Professor Claude E. Boyd, Ph.D., don’t expect the Mississippi native to slow down, despite the quiet announcement of his retirement earlier this month. The 50-year veteran researcher’s career has been truly extraordinary.
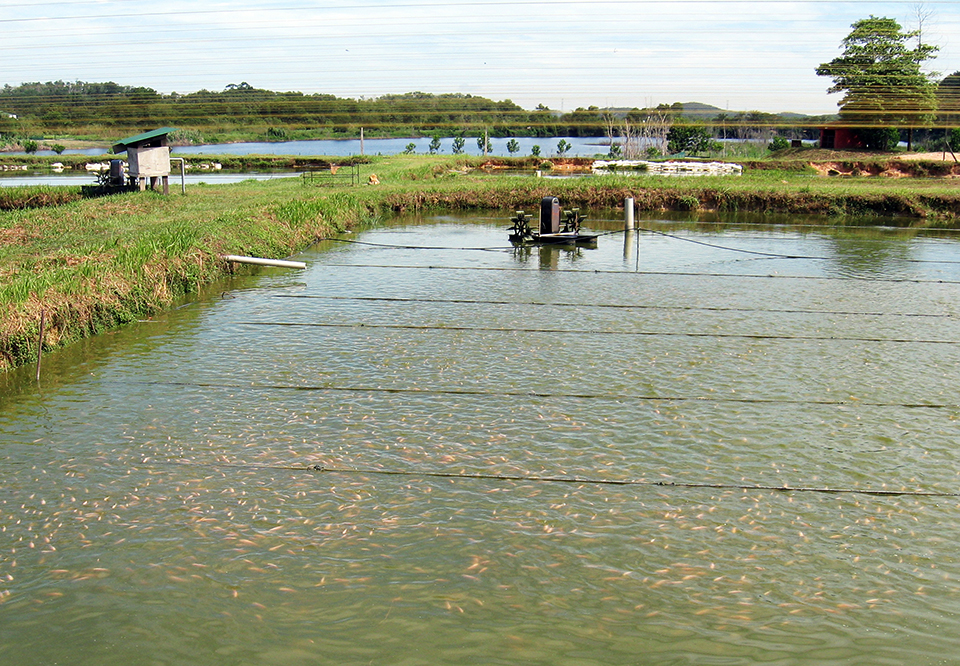
Responsibility
Assessing the carbon footprint of aquaculture
A carbon footprint is an estimate of the total carbon emissions resulting from the production, use and disposal of a product or service. Carbon footprints for aquaculture products result mainly from the use of manufactured feed and mechanical aeration.
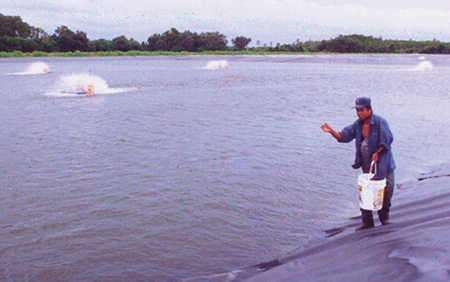
Aquafeeds
Feed study tests vegetable replacements for marine ingredients
Aquafeed study showed that an experimental diet with low levels of vegetable replacements for marine ingredients still produced acceptable results.
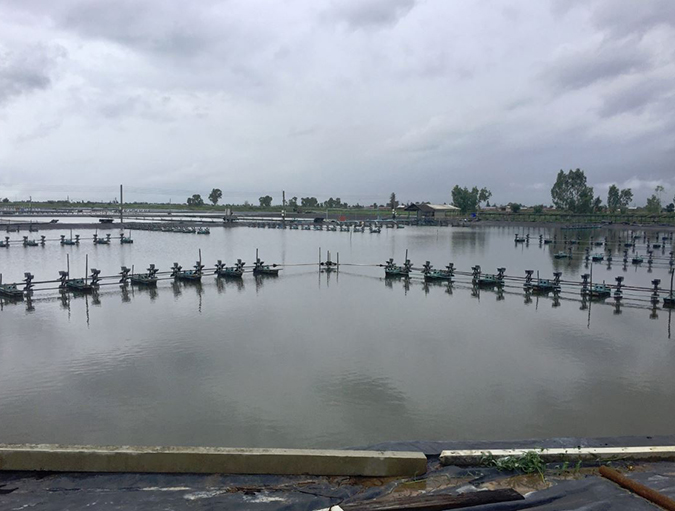
Health & Welfare
Aquamimicry: A revolutionary concept for shrimp farming
Aquamimicry simulates natural, estuarine production conditions by creating zooplankton blooms as supplemental nutrition to the cultured shrimp, and beneficial bacteria to maintain water quality. Better-quality shrimp can be produced at lower cost and in a more sustainable manner.