Experiments evaluate microcapsule density, mean volumetric particle size, water adsorption, buoyancy
One of the major, current challenges in aquaculture is the development of artificial diets and delivery systems that can effectively replace live feeds while adequately supporting development of the targeted organisms and providing economic and technological advantages. A recent study by the authors evaluated the physical and microstructural properties produced by a wall blend of whey protein-mesquite gum on an artificial diet encapsulated by spray drying, and determined the effects of a selected microcapsule formulation on the growth of penaeid shrimp larvae.
Microencapsulated diets
Diet microencapsulation using a biopolymer composite wall is a novel way to protect diet ingredients against deteriorative mechanisms such as oxidation and leaching. It also provides the diet with desired properties, such as particle size and buoyancy, so it is accessible to the organism for which it was designed.
Spray drying
The functionality of microencapsulated diets is dependent on the suitable selection of wall materials, which are highly dependent on the microencapsulation techniques used to apply them. Spray drying is a common and widespread method for drying materials that can also be employed for microencapsulating ingredients. The production of microcapsules by spray drying involves the drying of oil-in-water emulsions or solids-in-water dispersions.
Wall materials
Two potential biopolymers for use as wall materials in the encapsulation of aquatic larvae diets are whey protein (a milk protein) and mesquite gum (a polysaccharide exuded by the desert-growing mesquite tree). Previous studies by the authors and other researchers established that whey protein provides a good barrier against oxidation of encapsulated lipid materials. Mesquite gum provides essential oils with appropriate physical properties and microstructure, although it has low protein content.
The inclusion of a wall material of protein origin could provide microcapsules with higher protein content, and possibly interact with a polysaccharide wall material to allow enhanced microcapsule functionality.
Experimental setup
Table 1 lists the ingredients and percentages uses in formulating the experimental diets for zoea-stage Pacific white shrimp (Litopenaeus vannamei). These diets were encapsulated by spray drying using whey protein and mesquite gum in different proportions as wall materials.
Pedroza-Islas, Litopenaeus vannamei diet formulation, Table 1
Ingredients | Percentage (w/w) |
---|---|
Clam | 39.60 |
Squid | 24.90 |
Tuna flesh | 12.22 |
Crab pulp | 17.37 |
Sunflower oil | 0.45 |
Cod liver oil | 1.58 |
Corn starch | 3.28 |
Mineral premix | 0.06 |
Vitamin premix | 0.03 |
Vitamin C | 0.06 |
Soybean lecithin | 0.30 |
Cholesterol | 0.15 |
Four experiments to evaluate microcapsule density, mean volumetric particle size, water adsorption, and buoyancy were carried out, using 34 percent and 80 percent whey protein contents and wall material:diet ratios of 1:1 and 2:1. The morphology and internal microstructure of the microcapsules were determined by scanning electron microscopy.
The microcapsule with the best physical parameters and shape was selected for evaluation in a feeding assay with L. vannamei larvae. This diet (25 WP-80:75 MG) was compared with three commercial diets and a control live diet, microalgae Chaetoceros muelleri.
Larval shrimp culture was carried out in 1.5-l glass flasks placed in a controlled temperature water bath. Initial shrimp culture density was 100 nauplii per liter. A single dose of microalgae (60,000 cells per milliliter) was inoculated on the first day of the experiment. All diets were hydrated in seawater for three minutes and fed to the larvae twice daily at 8 a.m. and 8 p.m.
The shrimp larval stage was determined daily by visual examination. The experiment was terminated once animals reached the mysis substage. Surviving shrimp were counted, and their carapace lengths, individual dry matter weights, development indexes, metamorphosis indexes, and behavior indexes were determined.
Results
Microcapsule morphology and structure

All the microcapsules formed were of the microsphere type, with an internal void matrix as shown by Figs. 1 and 2. The whey protein content, whey protein-mesquite gum interaction, and wall material:diet ratio influenced the morphology and internal microstructure of the microcapsules.
Surface flaws were more evident in microcapsules with a material:diet ratio of 1:1 and 34 percent whey protein content. The microcapsules had a more spherical shape and smooth, uniform surface and internal structures with a biopolymer wall blend with 80 percent protein content.
Diet fat distribution was more uniform and homogeneous in the microcapsules with higher protein content. However, the proportion of mesquite gum in the blend was important in achieving better fat distribution and smaller particle size in the internal matrix. This was more noticeable with the biopolymer blend made of 25 percent whey protein and 75 percent mesquite gum, suggesting a synergy between the biopolymers evidenced by the more compact structure observed in Fig. 3.

Milk whey proteins are known to possess highly flexible lateral chains that contain polar and nonpolar moieties, the latter favoring their absorption at oil-water interfaces. On the other hand, the emulsifying properties of mesquite gum relate to its small protein content, covalently attached to the macromolecule polysaccharide hydrophilic chains that complement the interfacial action of the milk whey proteins.
Particle size
Mean volumetric particle size of the microcapsules varied widely at 3-40 mm. Variation was less when the wall material:diet ratio was 1:1 versus 2:1, possibly due to the thicker interfacial adsorbed layers formed around the diet particles, and in particular when 80 percent whey protein:mesquite gum (WP-80:MG) molecules interacted.
Buoyancy
The best microcapsule buoyancy was found with WP-80:MG in a 2:1 wall material:diet ratio. The characteristic floatability time constants fluctuated between 68.03 minutes for blend 75 WP-80:25 MG and 139.89 minutes for 25 WP-80:75 MG.
Microcapsule density
The density of the microcapsules varied 0.444-0.667 grams per cm3. The wall material:diet ratio did not influence microcapsule density.
Water absorption
The results indicated a variation between 8.11 (for blend 25 WP-80:75 MG, ratio 2:1) and 13.53 (50 WP-80:50 MG, ratio 2:1) g of water adsorbed per 100 g sample.
Bioassay
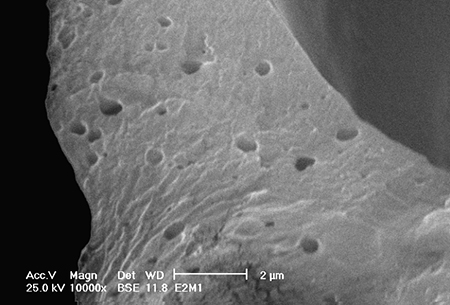
The microcapsule showing the best overall physical properties (mean volumetric particle size of 11.88 mm, density of 0.571 grams per cm3, buoyancy characteristic time 139.89) and morphological and microstructural properties was obtained with a 25 WP-80:75 MG blend in a wall material:diet ratio of 2:1. Selected for bioassay, its proximal composition was 16.32 percent crude protein, 3.54 percent crude fat, 3.73 percent crude fiber, 5.7 percent moisture, and 70.71 percent free nitrogen extract.
The bioassay results are presented in Table 2. The microencapsulated diet had an individual dry weight equivalent to the live control, and both were significantly higher than those of the three commercial diets. However, shrimp survival rate for the microencapsulated diet was 29.5 percent – equal to that of two commercial diets – while the live diet yielded 69.9 percent survival.
Carapace length measurements for the microencapsulated and commercial diets varied 0.72 to 0.81 mm, with no significant differences. Similar results were determined for the development and metamorphic indexes. The performance index varied 58 to 145.3. The highest value was shown by the live diet, followed by a commercial diet and the microencapsulated diet.
Pedroza-Islas, Results (mean value and SE) of larviculture test, Table 2
Feed | Individual Dry Weight (mg) | Survival (%) | Carapace Length (mm) | Development Index | Metamorphic Index (%) | Performance Index |
---|---|---|---|---|---|---|
25 WP-80:75 MG | 32.9 (5.8)a | 29.5 (3.6)a | 0.73 (0.0)ab | 3.3 (0.08)a | 32.3 (7.7)a | 76.9 (5.6)ab |
Commercial brand 1 | 26.4 (2.1)b | 73.2 (5.1)c | 0.72 (0.02)a | 3.5 (0.05)a | 50.3 (4.6)ab | 98.1 (4.6)bc |
Commercial brand 2 | 20.0 (0.0)c | 40.0 (4.9)ab | 0.76 (0.01)ab | 3.2 (0.03)a | 26.4 (1.4)a | 58.0 (4.0)a |
Commercial brand 3 | 20.4 (0.4)c | 41.8 (8.3)abc | 0.75 (0.04)ab | 3.1 (0.31)a | 24.6 (4.6)a | 68.6 (9.9)a |
Control | 33.3 (2.9)a | 69.6 (9.3)c | 0.81 (0.02)b | 4.2 (0.04)b | 96.8 (2.7)b | 145.3 (1.5)d |
Conclusion
The microencapsulation of artificial diets for shrimp larvae with biopolymer blends of whey protein-mesquite gum can provide functional feeds that compete favorably with commercial diets, but still fall short of the efficiency produced by live diets. Future work should contemplate the use of higher WP:MG proportions in the wall material blend, or the use of whey protein isolate (which has higher protein content) in conjunction with mesquite gum as the wall material.
Note: Cited references are available from the authors.
(Editor’s Note: This article was originally published in the December 2002 print edition of the Global Aquaculture Advocate.)
Now that you've reached the end of the article ...
… please consider supporting GSA’s mission to advance responsible seafood practices through education, advocacy and third-party assurances. The Advocate aims to document the evolution of responsible seafood practices and share the expansive knowledge of our vast network of contributors.
By becoming a Global Seafood Alliance member, you’re ensuring that all of the pre-competitive work we do through member benefits, resources and events can continue. Individual membership costs just $50 a year.
Not a GSA member? Join us.
Authors
-
Ruth Pedroza-Islas, Ph.D.
Departamento de Ingenierías
Universidad Iberoamericana.
Prol. Reforma 880 Santa Fe
01210 México, D.F. México[120,109,46,97,105,117,64,97,122,111,114,100,101,112,46,104,116,117,114]
-
Norma Espinoza-Herrera, M.S.
Departamento de Ingenierías
Universidad Iberoamericana.
Prol. Reforma 880 Santa Fe
01210 México, D.F. México -
Eduardo J. Vernon-Carter, Ph.D.
DIPH, Universidad Autónoma Metropolitana
Iztapalapa, México -
Carlos E. Medina-Reyna, M.S.
Universidad del Mar, Puerto Angel
Oaxaca, México -
Ivonne Santiago-Morales, M.S.
Universidad del Mar, Puerto Angel
Oaxaca, México -
Gabriela Gaxiola-Cortés, Ph.D.
Estación Experimental Ciudad del Carmen
Campeche, UNAM, México
Tagged With
Related Posts
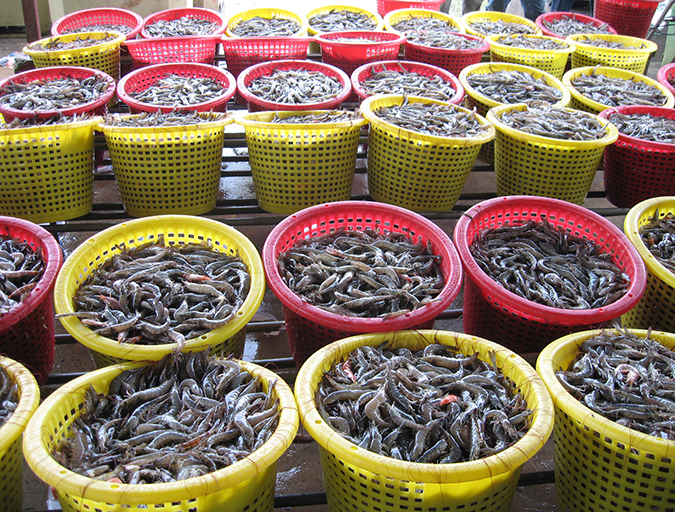
Aquafeeds
Microencapsulated organic acids aid shrimp culture
A novel blend of organic acids (OAB) microencapsulated in a lipid matrix and supplemented in the diets of Litopenaeus vannamei enhanced their growth, phosphorus utilization, resistance to Vibrio harveyi and immunity. This may have implications as an eco-friendly prophylactic strategy.
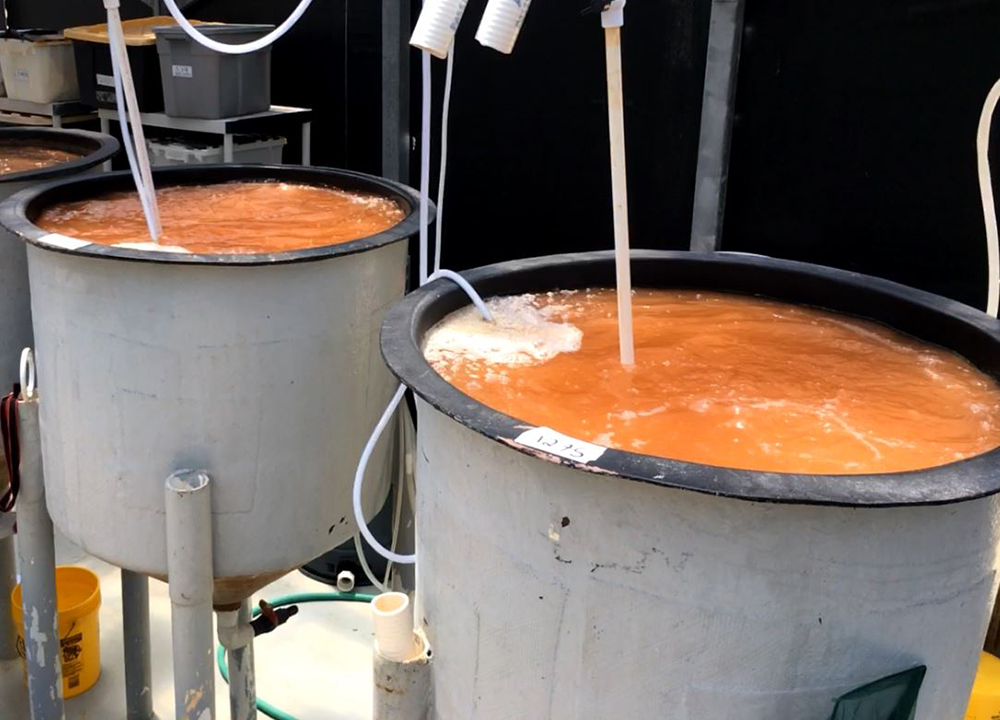
Aquafeeds
Global brine shrimp supply a potential bottleneck to aquaculture expansion, part 1
The brine shrimp artemia is critical for larval fish and shrimp diets. As aquaculture expands, an effective artificial replacement diet for artemia is a major consideration for the industry.
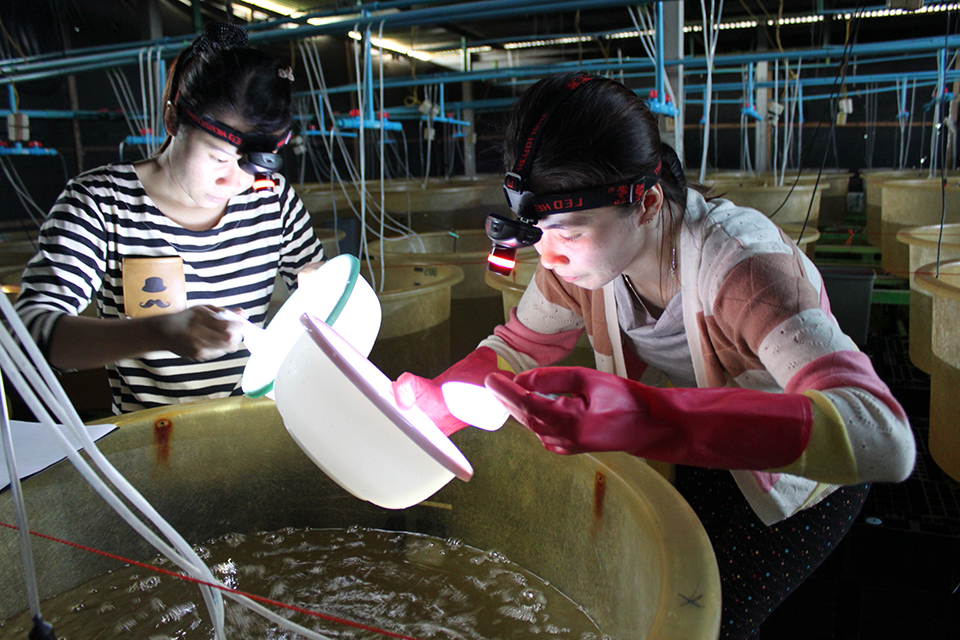
Health & Welfare
Maximizing profits of larval shrimp rearing
Easily digested, live artemia offer important animal health benefits and improved performance when included in feeding regimes for larval shrimp. Pilot and commercial-scale trials have confirmed that artemia can be partially replaced in shrimp feeding.
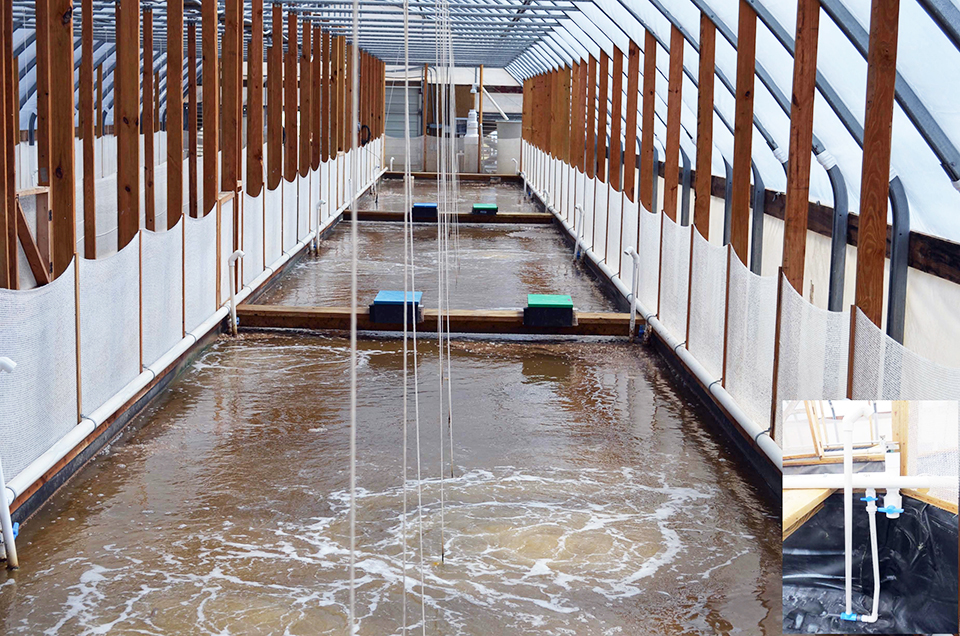
Health & Welfare
Nursery performance of Pacific white shrimp in zero-exchange biofloc systems
The authors conducted studies to test the performance of young shrimp postlarvae in systems with different approaches to aeration and water handling. Proactive management through proper feed applications and water quality control were critical elements.