Fish grew 12 percent faster and had a 17 percent better FCR in green water
Tilapia aquaculture in Brazil has surged over the last two decades, with cage culture in large reservoirs and intensified pond production. Enhanced aeration and water exchange have enabled farmers to harvest 40 to 120 metric tons (MT) of tilapia/ha/crop in earthen ponds, with higher stocking densities (7 to 15 fish per square meter) and feeding rates (600 to 1,400 kg/ha/day). Dense blue-green algae (cyanobacteria – BG) blooms require increased aeration (20 to 40 HP per hectare), cause issues regarding fillet off-flavor, and demand extensive use of algaecides and higher water exchange to control BG algae and maintain water quality.
Aeration costs rise as additional power is needed to keep up with BG algae’s oxygen consumption, often exceeding that of the fish. Many farms also rely on pumping for water exchange and adding to energy costs. High water exchange leads to nutrient-rich effluents polluting rivers or creeks that often are used by multiple farms for water collection and discharge, raising the risk of spreading harmful pathogens.
Environmental issues and conflicts with other water users will lead to more regulations on water use in aquaculture, increasing costs for settling basins and effluent treatments. Efficient water use is crucial for the sustainable growth of intensive pond production of tilapia and other fish in Brazil and globally. This article summarizes a production trial with new insights into sustainable intensive pond production.
Cyanobacteria issues in pond aquaculture
The management of BG algae blooms to counteract their effects on water quality and on the quality of fish is an important issue in intense pond production. Dense BG algae blooms lead to high water pH, and thus higher risk of ammonia toxicity; night-time depletion of oxygen and high carbon dioxide, suffocating fish; the release of microcystins and others cyanotoxins. Such negative effects negatively impact the well-being, growth performance and health of fish.
Water quality deterioration caused by dense BG blooms results in economic losses due to impaired growth and feed conversion, poor health and survival, intensive use of chemicals (algaecides), higher water exchange and increased aeration power to offset the increased oxygen demand by algae. More sustainable and effective strategies to control cyanobacteria bloom are needed rather than just reducing their densities by applying chemicals or exchanging more water.
Shading water using suspended clay
Shading is a way to prevent excessive growth of algae. Suspended clay particles can block sunlight and prevent the growth of microalgae, as farmers certainly have seen such effect in muddy ponds. Clay suspension would be a low-cost and easy-to-maintain strategy to block sunlight and prevent the problems caused by dense blooms of cyanobacteria, compared to other shading alternatives, such as artificial dyes and floating macrophytes.
Water can be intentionally turned muddy at the moment of filling the pond by turning on the aerators in shallow water areas. Only two negative aspects arise with the presence of suspended clay particles in intensive fish ponds: 1) the need to provide daytime intermittent aeration since there will not be enough photosynthesis to oxygenate the water (however, without the algae blooms, farmers could use less aeration power per hectare); 2) the lower availability of natural food, which may be a detrimental factor for filtering fish such as tilapia.
In the past, feeds available for tilapia were not nutritionally complete, and many farmers experienced poor growth of their tilapia in ponds with muddy water. However, in the last twenty years, with the expansion of tilapia farming in cages and other intensive systems, the knowledge on tilapia nutrition has increased substantially and high-quality feeds have become available, allowing for tilapia growth and feed conversion ratios (FCR) in cages to match the values observed in ponds. For other fish species for which the presence of natural food plays minor importance, such as channel catfish and other non-planktivorous fish, growth and FCR should not be significantly affected in muddy ponds.
A trial to compare intensive tilapia production in green vs muddy waters
What would be the benefits and drawbacks of using suspended clay to get the phytoplankton out of the pond equation? 1. Less water exchange? Without algae photosynthesis, afternoon water pH stays at around 7; at such pH level the risk of ammonia toxicity is low. There could be up to 30 mg per liter of total ammonia in the water and the toxic ammonia would still be at safe levels for the fish (less than 0,2 mg per liter), and then water exchange to dilute ammonia could be less frequent; 2) Reduce issues with fillet off-flavor? Without BG algae, there would be less geosmin (GMB) and methyl-isoborneol (MIB) in the water – the cause of off-flavor – minimizing this issue. 3) Lower growth and feed efficiency? With no extra nutrition coming from phytoplankton, growth and feed efficiency could be impaired; 4) Lower energy cost for aeration? As the main oxygen consumer (phytoplankton) would be removed, aeration power could be reduced.
A small-scale experiment was conducted to verify the production performance, water quality, water use, and off-flavor intensity for tilapia cultured in green or muddy water. Sixteen 1.02 square meter tanks were used, with eight managed with green water (phytoplankton) and eight with muddy water (suspended clay). Green water tanks had a substrate of 42 kg of sand and 2 kg of dry soil, while muddy water tanks had 44 kg of dry soil. Soil was collected from an empty pond, sun-dried, and mixed before application. Tanks were filled with 850 liters of green water with an initial transparency of 47 cm, pH 7.7, total alkalinity 18 mg CaCO3 per liter, and total hardness 21 mg CaCO3 per liter. The initial water tested zero for ammonia and nitrite and had 40 mg per liter of nitrate. Each tank received 300 grams of salt to prevent nitrite intoxication and 8 grams of hydrated lime to raise total alkalinity above 30 mg CaCO3 per liter.
Twelve 284-gram all-male Nile tilapia juveniles were stocked in each tank. Diffused air was provided using a 20-cm Aerotube® hose. In muddy ponds, the air diffuser was placed at the bottom to suspend clay particles on the first day, then repositioned at 25 cm below the water surface. In green water ponds, air diffusers were always kept 25 cm below the surface. Fish were fed two to three times daily with commercial floating feed. Key water parameters were monitored, and water exchange was performed always that toxic ammonia levels reached 0.5 mg per liter or above.
The volume of water exchanged and any make-up water added were recorded for each tank. Additional hydrated lime additions were made as needed to maintain water alkalinity above 30 mg CaCO3/L. The experiment lasted 92 days, after which fish were weighed, killed in ice-cold water, and filleted for sensory tests to detect off-flavor intensity.
Production results and water use
After 92 days, the tilapia grew from the initial 284 grams to a mean weight of 665 grams in the muddy water tanks, and to 753 grams (13 percent higher) in the green water tanks. The FCR was 1.72 in green water (17 percent better) compared to 2.01 in muddy water. No dead fish were observed. However, at the end of the experiment, two green water tanks were lacking one fish each. These fish probably jumped out, despite the bird net protection. Nonetheless, average survival was above 98 percent and was not different in the green or muddy water tanks.
Kubitza, Muddy ponds, Table 1
Parameters recorded | Green water | Muddy water |
---|---|---|
Mean initial weight (g) | 284 | 284 |
Mean final weight (g) | 753 | 665 |
Avg. daily weight gain (g/d/fish) | 5.1 | 4.15 |
Initial standing crop (kg/m2) | 3.34 | 3.34 |
Final standing crop (kg/m2) | 8.77 | 7.82 |
Avg. total feed applied (kg/tank) | 9.36 | 9.01 |
FCR | 1.72 | 2.01 |
Survival (percent) | 99.0 percent | 100.0 percent |
Total water use (liters/tank) | 5.338 | 1.003 |
Avg. Daily water addition (percent tank volume) | 5,8 percent | 0,2 percent |
Mean water use per kilo of fish harvested | 978,5 | 223,8 |
The average water use was 1,003 liters per tank with muddy water compared to 5,338 liters per tank with green water (Table 1), including the initial filling of the tanks with 850 liters. In tanks with muddy water, we mostly replaced the volume lost through evaporation (almost zero renewal). However, water exchanges were often required in green water tanks to maintain toxic ammonia levels below 0.5 mg NH3 per liter. On average, green water tanks received a water input of 5.8 percent of tank volume per day, compared to only 0.2 percent in muddy water tanks. Overall water use per kilogram of biomass gain was 4 times higher in green than in mud water (979 liters per kg vs. 224 liters per kg, respectively).
Mean final standing crop was 7.8 kg per square meter in muddy water tanks, and 8.8 kg per square meter in green water tanks (12.1 percent higher; Table 1), corresponding to 78 and 88 MT/ha, respectively. These values equal the tilapia biomass harvested by Brazilian farmers from intensively managed green water ponds with aeration at 30 to 40 HP per hectare and high water exchange (up to 30 percent per day during the final weeks of culture). Feeding rates were similar in both environments and ranged from 70 to 130 grams per square meter per day from the beginning to the end of the experiment. This is equivalent to 700 to 1,300 kg of feed per hectare per day (Fig. 5a).
The better growth and FCR for tilapia in green water tanks were likely due to microalgae supplementing marginal nutrients in their feed. In the last four weeks of the trial, tilapia in muddy water tanks took longer to eat and often left pellets uneaten. As muddy water tanks received less water exchange, it is possible that an undetected water quality issue may have caused discomfort to the fish, reducing feeding activity and impairing growth and feed efficiency.
Water quality
Water temperatures ranged between 23 and 27 degrees-C at 7 a.m. and between 26 and 33 degrees-C at 2 p.m. Dissolved oxygen ranged from 2.8 to 7.3 mg per liter in muddy water and from 3.2 to 7.6 mg per liter in green water (Fig. 1a,b). No significant differences in these parameters were observed between the two environments.
Water pH at 3 pm remained relatively stable in muddy water (7.5 to 8.3), compared to 6.5 to 8.0 in green water. For the first five weeks, green waters had higher pH than muddy waters, with values decreasing thereafter, most likely due to the intense respiration of microalgae and fish (Fig. 2a). Frequent water exchanges in green ponds helped to keep microalgae controlled, moderating afternoon pH.
Total alkalinity was managed to keep it above 30 mg CaCO3 per liter with the addition of hydrated lime as needed. In the last 8 weeks, water exchange was intense in the green water tanks, causing the average total alkalinity value to fall just below 20 ppm. At the same time, average total alkalinity increased in the muddy water tanks, mainly in response to the increase in total ammonia. Ammonia is, chemically, a base and thus it is titrated in the alkalinity test (Fig. 2b).
Carbon dioxide levels at 7 a.m. were significantly higher in green water (12 to 26 mg per liter) than in muddy water (5 to 10 mg per liter), mainly due to microalgae respiration (Fig. 3). Carbon dioxide levels were three times higher in green vs. muddy water tanks. Because carbon dioxide generation is proportional to the oxygen consumption, in a commercial production setting the aeration power required is expected to be higher in green water ponds.
Regarding nitrogen metabolites, even with an intense water exchange, total ammonia values were higher in green water tanks (10-20 mg per liter) than in muddy water tanks (below 5 mg per liter, as shown in Fig. 4a). Toxic ammonia levels only reached 0.5 mg NH3 per liter in some muddy water tanks in the last two weeks of the trial (Fig. 4b). Nitrite concentration started higher in muddy (10 mg per liter) than in green water tanks (0 mg per liter; Fig. 4c), certainly due to the presence of nitrite and nitrate in the pond soil used as a substrate. Muddy water tanks started with 44 kg of pond soil, while green water ponds with 42 kg of sand and only 2 kg of pond soil. In muddy water, nitrite and ammonia peaked on day 6 (30 and 8 mg per liter, respectively) and then declined. In green water tanks, nitrite increased from day 6, peaking at 60 mg per liter on days 16-19, one week after the total ammonia peak (22 mg per liter NH3), and then declined below 10 mg per liter. Such high nitrite levels apparently did not cause major discomfort to the fish, probably due to the application of sodium chloride which minimized the nitrite poisoning risk.
The low levels of total ammonia and nitrite in muddy water tanks, despite the high feeding rates (700 to 1,400 kg of feed per hectare, Fig. 5a) are attributed to the high activity of nitrifying bacteria colonizing the suspended clay particles, transforming the entire water column into a powerful biofilter. This made it possible to sustain a standing crop of tilapia near 80 MT per hectare in an earthen pond environment with almost zero water exchange.
Concerning settleable solids, the water used to fill the tanks had practically no solids. In green water tanks, the suspended solids initially consisted of microalgae. In the first 4 weeks, the tanks with muddy water had more settleable solids (up to 10 mL per liter) than the green water ones (Fig. 5b) due to the intentional suspension of clay on the first day after filling. As the experiment progressed, there was a considerable increase in organic particles, especially fragments of fish feces, which made the settleable solids gradually increase, reaching values between 15 and 20 mL per liter in the last weeks of the trial. From day 30 until the end, the concentrations of settleable solids were practically the same in both the green and muddy water tanks.
Water transparency was quickly reduced from the initial 47 cm to 12 cm in muddy water tanks, with the intentional suspension of clay on day 1. Water transparency in muddy water remained between 4 and 10 cm throughout the experiment (Fig. 5c). In green water tanks, water transparency gradually declined from the initial 47 cm to values close to 10 cm. This reduction in transparency occurred due to the increase of organic particles suspended in the water, especially microalgae (phytoplankton) and fecal fragments.
Regarding off-flavor issues, frequent water exchange helped to maintain moderate algae blooms, which certainly reduced off-flavor intensity in the fillets of tilapia from green water tanks. Nonetheless, the fillets of tilapia raised in muddy water, with nearly zero water exchange, had half of the off-flavor intensity perceived in the fillet of fish raised in green water (Table 2). Over many years of field work, I have observed that fish from muddy water ponds seldom had off-flavors. Clay particles reduce light intensity in the water column, and thus, the growth of cyanobacteria. With this, the concentration of off-flavor compounds in the water should be minimal. Clay particles may also be able to adsorb off-flavor compounds in the water, preventing the fish uptake through their gills.
Kubitza, Muddy ponds, Table 2
Culture environment | Off-flavor degree in tilapia fillet |
---|---|
Muddy water | 0,625* |
Green water | 1,375 |
Off-flavor score | Off-flavor condition |
0 | No odor or taste is noticed in a cooked fillet sample. |
1 | Off-flavor is almost imperceptible when tasting a cooked fillet sample. |
2 | Off-flavor is detected only after tasting a cooked fillet sample. |
3 | Off-flavor is already detected in the odor of a cooked fillet sample. |
4 | Off-flavor detected in the odor of a raw fillet sample. |
Final remarks
To my knowledge, this is the first study comparing tilapia performance, water quality and off-flavor intensity in green and muddy waters. Tilapia grew 12 percent faster and had a 17 percent better FCR in green water, likely due to additional nutrition from microalgae and possibly better water quality. By improving feed and water quality management in muddy waters, these differences in growth and FCR can be reduced.
Supporting high fish yield with almost zero water exchange and lower off-flavor intensity brings to light the perspective of high-density pond culture of tilapia in muddy water, as well as of other farmed fish like channel catfish (Ictalurus punctatus), tambaqui (Colossoma macropomum), pangasius and other species. Intermittent aeration, though, will be needed in muddy water ponds, but without the dense BG algae blooms, the aeration power can be reduced to 50 percent or less of what is needed in green water ponds, offsetting the extra hours of aeration required. This deserves verification in a larger-scale pond experiment.
This definite reduction in water use and effluent discharge supports the sustainable growth of intensive pond aquaculture worldwide, especially in water-limited regions. Further research and development are needed on the management of muddy water ponds, warranting attention from producers, industry, and researchers.
Now that you've reached the end of the article ...
… please consider supporting GSA’s mission to advance responsible seafood practices through education, advocacy and third-party assurances. The Advocate aims to document the evolution of responsible seafood practices and share the expansive knowledge of our vast network of contributors.
By becoming a Global Seafood Alliance member, you’re ensuring that all of the pre-competitive work we do through member benefits, resources and events can continue. Individual membership costs just $50 a year.
Not a GSA member? Join us.
Author
-
Fernando Kubitza, Ph.D.
Jundiaí, São Paulo, Brazil
Dr. Fernando Kubitza is a Brazilian Aquaculture specialist, founder and director of Acqua Imagem Services in Aquaculture (www.acquaimagem.com.br), a 25-year-old company leading on aquaculture projects, training and technical support in Brazil.[114,98,46,109,111,99,46,109,101,103,97,109,105,97,117,113,99,97,64,111,100,110,97,110,114,101,102]
Related Posts
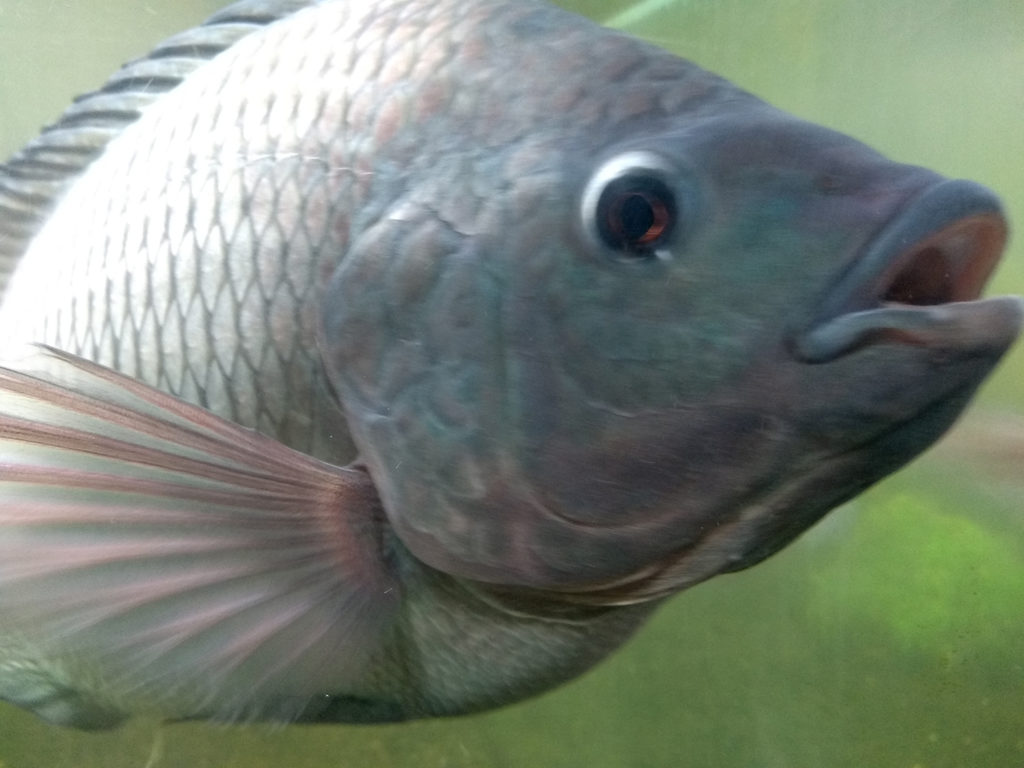
Health & Welfare
Advances in tilapia nutrition, part 1
This two-part review brings together scientific and field advances in tilapia nutrition and feeding to better support the formulation of feeds for better production performance.
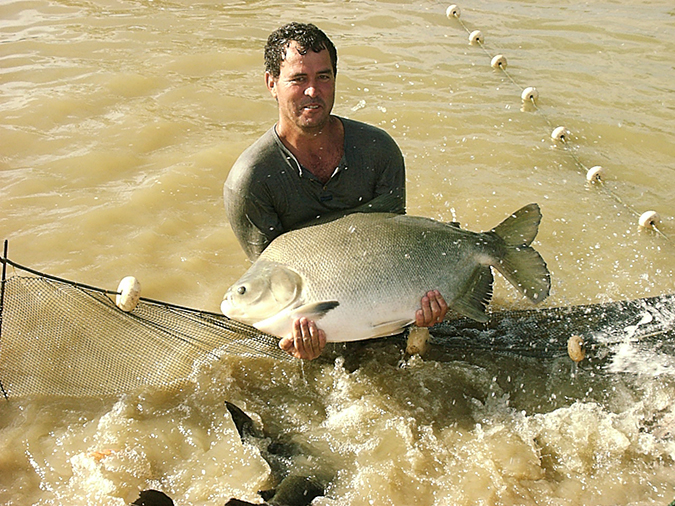
Intelligence
Brazilian aquaculture: Constraints and challenges (Part 1)
The Brazilian aquaculture industry has been growing steadily during the last two decades. Despite facing a number of challenges it is looking at continued growth and a larger role in the export markets.
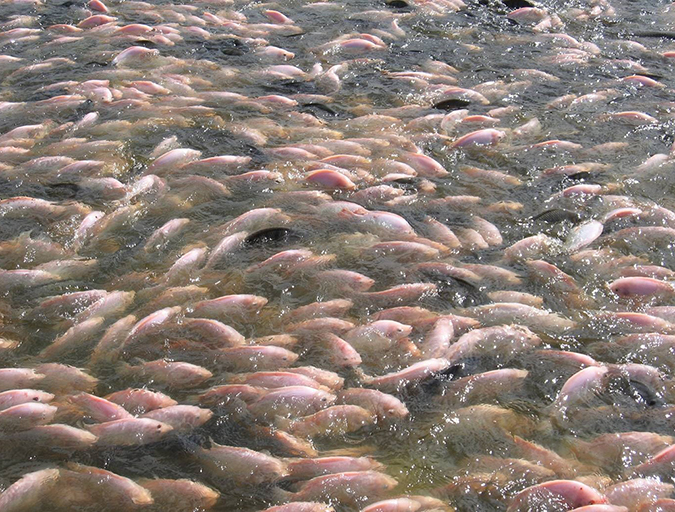
Health & Welfare
Common salt a useful tool in aquaculture, part 1
The preventive use of common salt (sodium chloride) by commercial producers of freshwater fishes has many benefits, including helping with the routine prevention of losses due to diseases, stress and mishandling during transport, harvesting, grading, counting, weighing and induced spawning.
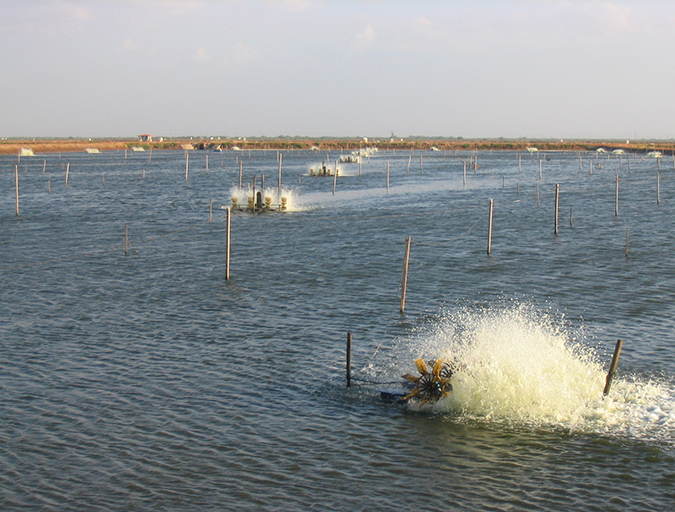
Health & Welfare
Proper water circulation in aquaculture ponds critical
Promoting water circulation during the day time is an effective strategy to enrich pond water with oxygen produced by photosynthesis, and can significantly reduce the costs of night time supplemental aeration.