Nutrient tranformation and water quality benefits
The challenge facing aquaculture nutritionists is to continually reduce feed costs, improve conversion efficiency, and minimize environmental impact. The conventional approach is to more accurately define the nutrient requirements of the target animal, the nutritional value of feed ingredients, and the impact of feed processing.
However, one factor that is often overlooked is the nutritional contribution of the culture environment. In the case of rainbow trout raised in fast-flowing spring water, this contribution may be negligible. But in systems with limited or no water exchange and a heavy organic load, such as shrimp or tilapia ponds, the contribution can be substantial.
Ecology of organic detritus
Major advances in understanding nutrient pathways in aquaculture systems resulted from early studies of natural ecosystems. For example, ecological modeling of mangrove estuaries traced the mysterious pathway by which mangrove leaf litter with a protein level of 3 to 6 percent was transformed into highly nutritious food called “organic detritus.”
Detritus refers to disintegrated or eroded matter. The term organic detritus was proposed by Odum and de la Cruz (1963) to designate particulate organic material originating from the dead bodies, nonliving fragments, and excretions of living organisms. Modeling studies delineated the mechanisms by which organic material is incorporated into the aquatic ecosystem.
Particles of decomposing organic material, which are poor in essential nutrients, are coated with a film of bacteria. The bacteria absorb nutrients from the water and the organic particles. This process decreases the energy of detrital particles, but increases their protein and phosphorus levels, thereby raising their food value (Wood-house and Knutson, 1982).
Role of detritus
Organic detritus plays a major role as a food source for a variety of estuarine organisms, including amphipods, fiddler crabs, snails, mussels and juvenile fish. The film of bacteria and other microorganisms generally constitutes 5-10 percent of the mass by weight of detrital particles. Detritus-feeding organisms derive nourishment by strip-ping the microorganisms from the detrital material as it passes through their guts.
The fecal pellets may be recolonized and the process repeated until all the organic material has been utilized. By taking advantage of the recy-cling capability of organic detritus within the culture environment, nutritionists and pond managers can reduce feed costs, improve conversion efficiency, and reduce environmental impact.
Characteristics of intensive microbial reuse systems
Intensive aquaculture systems historically have relied on high rates of water exchange to flush out wastes and maintain water quality. Increasing demand for water conservation, restrictions on effluent disposal, and need for biosecurity are now limiting the use of flow-through systems.
An alternative waste treatment is intense microbial processing of wastes to facilitate high yields with little or no water exchange. Yields in such aerated microbial reuse (AMR) systems have ranged from 1 to 2.6 kilogram per square meter for shrimp, and from 10 to 100 kilogram per square meter for tilapia.
The concept of AMR systems is simple: Potentially toxic nitrogenous wastes are converted into bacterial protein, which is then available for detrital feeders. AMR requires strong aeration and mixing to continually suspend organic waste in the water column for digestion by bacteria. Aerobic heterotrophic bacteria colonize the particles of organic waste and absorb nitrogen, phosphorus, and other nutrients from the water. This process improves water quality and recycles wastes as bacterially enriched detritus. Typically, no external filtration and little or no solids removal is required.
Accumulation of ammonia
In conventional culture systems, only about 20 to 30 percent of the carbon, nitrogen, and phosphorus in feeds is assimilated by fish and shrimp. The remainder is lost as uneaten feed and excretory waste. Much of the nitrogen is in the form of ammonia resulting from protein deamination. Unionized ammonia is toxic to aquatic animals.
In semi-intensive systems, ammonia accumulation is seldom a problem, due to its absorption by phytoplankton and bacteria. However, in intensive systems, increasing levels of ammonia ultimately stress animals, suppress growth, and limit yields. To prevent ammonia toxicity, interventions such as water exchange or external nitrification is introduced.
An alternative is AMR, which encourages the development of heterotrophic bacterial communities within the culture system through strong mixing, aeration, and the addition of carbonaceous substrates.
Carbon to nitrogen ratio
Heterotrophic bacteria have the remarkable ability to synthesize protein from organic carbon and ammonia. However, it is crucial that the ratio of carbon to nitrogen (C:N) be suitable for bacterial utilization. As most gardeners know, bacteria are inefficient at composting organic materials that are either too carbonaceous (leaves or wood), or too nitrogenous (high-protein vegetable meals or manures).
Balanced mixtures of carbonace-ous and nitrogenous materials with C:N of approximately 20:1 are more readily digested. For example, cellulose is a highly carbonaceous material resistant to bacterial decomposition. But Avnimelech et al (1999) demonstrated that the combination of cellulose powder and ammonium sulfate produced sufficient bacterial protein to grow unfed tilapia at one-third their normal rate.
Supplemental organic carbon
In practice, most aquaculture diets are designed for the target organism without consideration of the culture environment. As a result, C:N is generally deficient, and production systems accumulate inorganic nitrogen.
Provision of supplemental organic carbon to balance C:N allows bacteria to utilize ammonia to produce bacterial protein. This process improves water quality and creates a supplemental protein source. Avnimelech et al. (1994) demonstrated that the addition of wheat flour (a source of carbon) to the surface of super-intensive tilapia tanks reduced ammonia levels and improved food conversion.
Solar aquafarms experience
Steven Serfling, former CEO of the Solar Aquafarms indoor AMR tilapia farm in California, USA, described the tendency of inexperienced technicians at the facility to replace a portion of the “dirty” bacterial floc water with clean water. This would reduce the nitrogen-digesting capability of the system and cause increases in ammonia levels.
As ammonia levels rose, inexperienced technicians would typically respond by replacing more water, which would further exacerbate the situation. Serfling’s solution in such a crisis was the addition of carbonaceous material, such as wheat flour or alfalfa, to provide a source of organic carbon for bacteria to digest the ammonia.
Calculations
Avnimelech (1999) presented formulas for calculating the amount of organic carbon required to reduce inorganic nitrogen levels. To reduce ammonia levels in the culture system, he suggested that carbohydrate be added at 20 times the level of ammonia N. For example, if ammonia levels must be reduced by 1 grams per cubic meter, then 20 grams per cubic meter of carbohydrate should be added to the culture system.
Organic carbon sources
Sources of organic carbon include alcohols, sugars, starches, and fiber. Alcohols and sugars are highly digestible. This can be an advantage in initially establishing a bacterial population to compete with phytoplankton for absorption of nitrogen and phosphorus within a pond
Complex carbohydrates from grains such as corn and wheat are metabolized more slowly. They have the advantage of providing a particulate structure for the attachment of bacteria, as well as a more sustained release of organic carbon. In addition, they require a suite of bacterial enzymes for decomposition. These enzymes may improve digestion in the target aquaculture species when it consumes the detrital material.
Fibrous materials are generally avoided, because they are relatively resistant to decomposition. However, they may have some utility in providing a long-term structure for the attachment of bacteria.
System modeling
Models are being developed to predict carbon and nitrogen accumulation and oxygen requirement in detrital ponds. Avnimelech et al (1995) described the degradation rate for organic carbon (C) by microorganisms with a first-order kinetic expression:
Avnimelech found that the rate constant for organic carbon degradation in simulated and actual intensive fish ponds with constant aeration and mixing was 0.15 per day. At this rate, 90 percent of a feed addition of 500 kilograms per hectare would be degraded within 15 days. Organic nitrogen degradation was also evaluated using the above equation. The relevant rate constant for organic nitrogen degradation was 0.06 per day.
Montoya et al. (1999, 2000) developed a simulation model to examine feed and feeding strategies of nitrogen and phosphorus dynamics in AMR systems that allow daily predictions of:
- inorganic nitrogen forms (ammonia, nitrite and nitrate)
- dissolved reactive phosphorus
- shrimp growth and survival.
A carbon compartment is currently being developed to more accurately represent bacterial and phytoplankton dynamics within AMR systems.
Community succession
A process of community succession occurs over time within AMR ponds in response to the increasing organic load and maturity of the ecosystem. For example, in intensive shrimp ponds in Belize that are filled with clear Caribbean seawater and operated with strong aeration and zero water exchange, the following phases have been observed:
- Clear water.
- Algal bloom.
- Large amount of foam on the surface of ponds, due to accumulating dissolved organic material and inadequate bacterial community.
- Change to brown water color, disappearance of foam, and generation of flocculated masses of bacterial cells, organic detritus, and adsorbed colloids.
Floc masses
Floc particles are agglutinated by a polysaccharide slime produced by bacteria. Suspended materials are adsorbed onto the floc, where they are hydrolyzed by extracellular bacterial enzymes.
Older ponds are characterized by large floc masses that rapidly settle out of suspension if water agitation is stopped. AMR systems at this stage are highly stable and can process large amounts of organic inputs. McIntosh (1999) used feeding levels as high as 450 kilograms per hectare day with no water exchange. The volume of the flocs constitutes about 1 to 3 percent of the water volume, thus they can be easily filtered out of the pond.
Aeration and mixing
In most aquaculture systems, the oxygen content of the water is governed more by the activities of algae and bacteria than the target culture species. As conventionally managed ponds reduce or eliminate water exchange, higher loads of suspended organic material and bacteria accumulate in the water.
Aeration rates
As a result, biological oxygen demand increases, and aeration rates must be increased accordingly. For example, Hopkins et al. (1993) reported that 20 horsepower per hectare of aeration was sufficient in zero-water-exchange ponds with production rates of 3.2 metric tons (MT) per hectare, but mass mortality occurred when the same conditions were used to attempt 6 MT per hectare.
McIntosh (1999, 2000a) reported aeration requirements for AMR shrimp ponds in the range of 1 hp for each 300 to 550 kg of production in zero-water-exchange systems producing 11 to 26 MT per hectare cycle. The upper end of this range was reached by periodically draining concentrated organic sludge from a point of accumulation near the center drain.
Water mixing
In addition to oxygenation, aerators must provide sufficient mixing to prevent anaerobic zones of sedimented sludge. Anaerobic sediments generate toxic waste products such as nitrite, hydrogen sulfide, and methane. This is particularly important in seawater systems, where the abundance of sulfate favors the production of hydrogen sulfide under anaerobic conditions.
McIntosh (2000a) reported that water velocities of 10 to 20 centimeters per second are required to keep organic material in suspension in his intensive shrimp ponds. Toward the middle of the ponds, where velocity drops below 10 centimeters per second, solids begin to fall out of suspension.
Note: Cited references are available on request from the authors.
(Editor’s Note: This article was originally published in the April 2001 print edition of the Global Aquaculture Advocate.)
Now that you've reached the end of the article ...
… please consider supporting GSA’s mission to advance responsible seafood practices through education, advocacy and third-party assurances. The Advocate aims to document the evolution of responsible seafood practices and share the expansive knowledge of our vast network of contributors.
By becoming a Global Seafood Alliance member, you’re ensuring that all of the pre-competitive work we do through member benefits, resources and events can continue. Individual membership costs just $50 a year.
Not a GSA member? Join us.
Authors
-
George Chamberlain, Ph.D.
Global Aquaculture Alliance
St. Louis, Missouri, USA[103,114,111,46,101,99,110,97,105,108,108,97,101,114,117,116,108,117,99,97,117,113,97,64,110,105,97,108,114,101,98,109,97,104,99,46,101,103,114,111,101,103]
-
Yoram Avnimelech, Ph.D.
Faculty of Agricultural Engineering Technion
Israel Institute of Technology
Haifa, Israel -
Robins P. McIntosh
Belize Aquaculture, Ltd.
Belize City, Belize -
Mario Velasco, Ph.D.
Empacadora Nacional C.A.
Guayaquil, Ecuador
Tagged With
Related Posts
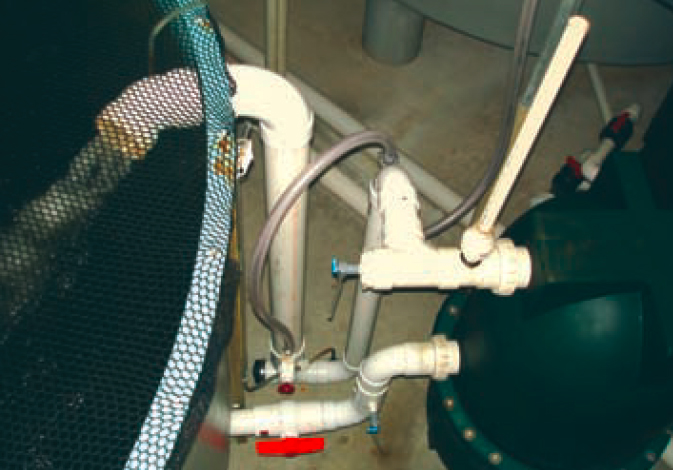
Responsibility
Airlifts combine pumping, water treatment in recirculation systems
Using the buoyancy of the entrained air bubbles to lift the water, airlifts are more energy-efficient and provide more aeration, carbon dioxide removal and foam fractionation for dissolved solids removal than centrifugal pump systems.
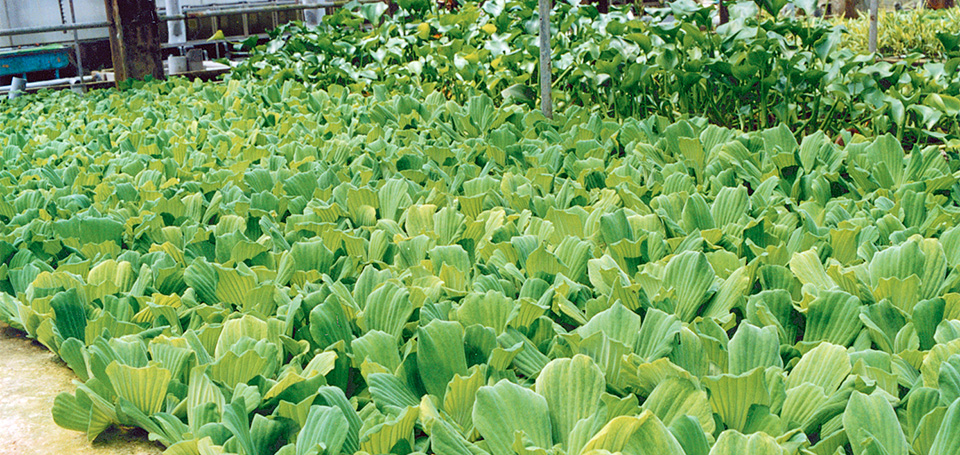
Responsibility
Denitrification in recirculating aquaculture systems: Comparison of aquatic plants
Denitrification by anaerobic bacteria can remove nitrates from RAS, but the operation requires anaerobic conditions and the addition of carbon sources.
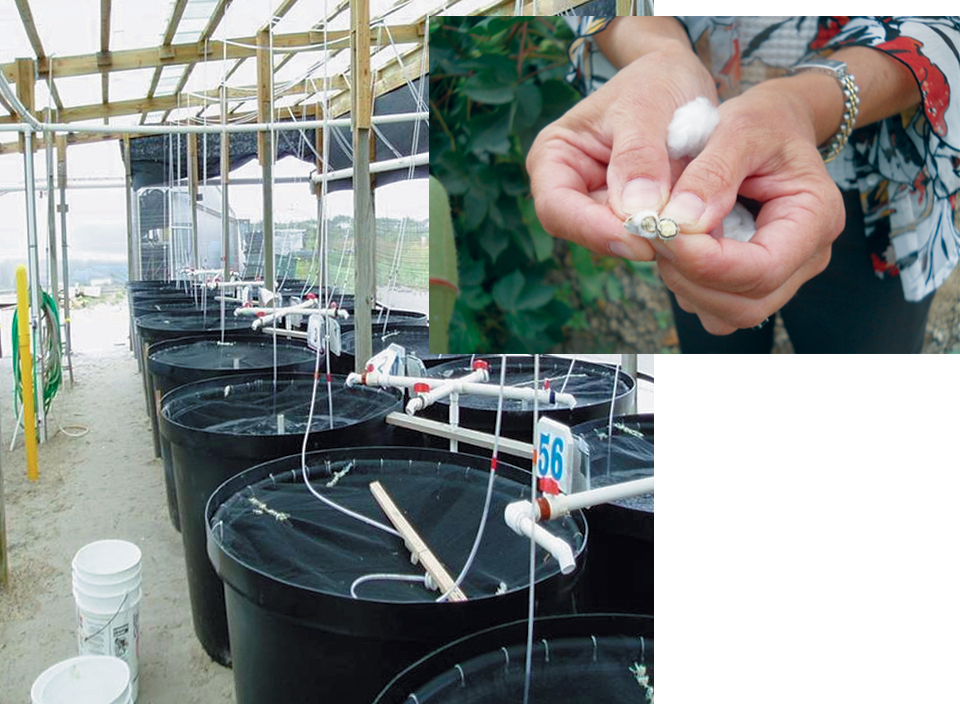
Aquafeeds
Glandless cottonseed meal replaces fishmeal in shrimp diet research
Cottonseed meal is high in protein and less expensive than fishmeal and soybean meal. Cotton plants can be engineered without gossypol in their seeds.
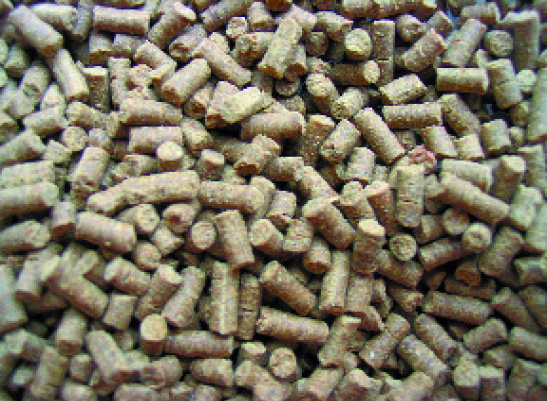
Health & Welfare
Protein-energy ratio research in penaeid shrimp
Of all the issues important to shrimp nutrition, the reduction of feed cost with concomitant increased feed performance are most desirable.