Bacteriophage therapy needs more understanding of the susceptibility of virulent bacterial strains, their genetic traits involved in pathogenicity and phage-bacterium co-evolution

Bacteriophages are viruses that specifically target bacteria and, therefore, can be used to kill bacteria without harming animal cells. As such, they represent an attractive alternative to antibiotics in treating bacterial infections in both humans and animals. Bacteriophages vary greatly in size, morphology, genome, host specificity, and strain tropism. Depending on their replication strategy, bacteriophages are classified as lytic (virulent) or lysogenic (temperate). Lytic phages hijack bacterial cell machinery upon entry, rapidly replicate, and cause cell lysis, releasing hundreds of infectious viral particles ready to infect other host cells (productive infection). In contrast, lysogenic phages stably integrate their genome into the bacterial chromosome, persisting latently and replicating along with the bacterial chromosome.
Phage therapy offers advantages over antibiotics, such as self-replication (phages multiply in the presence of bacterial targets, allowing for smaller doses and lower costs), auto-dosing (phages disappear upon target bacteria elimination), lack of interference with non-target bacteria or body cells (narrow action spectrum), and high strain specificity. However, the latter feature may be a disadvantage, which can be managed by using bacteriophage cocktails (mixtures of different phages). Additionally, phages are more environmentally friendly than antibiotics, can clear biofilms too, and, due to their short development cycles, are low-cost and easy to use and store.
Phages co-evolve with their hosts, and counter-adaptation to developed resistance is likely to occur, ultimately benefiting bacteriophage therapy in the long term. To improve bacteriophage therapy, it is essential to expand the understanding of phages, particularly through the annotation of complete DNA genome sequences for accurate identification, prediction of transduction efficiency, and avoidance of undesirable elements. Additionally, it is also necessary to clarify the mechanisms behind the emergence of phage resistance, which are still largely unknown.
Bacteriophages were used against bacterial diseases in past centuries, during the pre-antibiotic era, although their nature was unclear. Western countries abandoned their use with the introduction of antibiotics, and it is only in recent years that they have been rediscovered and used in regulated clinical trials, well-documented in the scientific literature. This rediscovery was driven by the urgent need for alternative therapies due to the rise of clinically relevant multi-antibiotic resistance.
This article – summarized and adapted from the original publication (Albarella, D. et al. 2025. Bacteriophage Therapy in Freshwater and Saltwater Aquaculture Species. Microorganisms 2025, 13(4), 831) – discusses a review of the latest advancements in developing and applying bacteriophage therapy to treat the most common and economically impactful bacterial diseases in freshwater and saltwater aquaculture species.
Phage therapy in aquaculture
Bacterial diseases are the major challenge in aquaculture, resulting in huge economic losses. Aquatic animals are susceptible to numerous bacterial infections or co-infections, as they are exposed to a wide variety of pathogenic microorganisms in their environment.
In the absence of effective vaccines that could prevent the majority of diseases affecting aquatic species, or due to the practical impossibility of vaccinating fish at early life stages because of their small size or immature immune system, phage therapy plays the dual role of therapeutic and preventive measures against bacterial infections in aquaculture. This is especially important during larvae production, before the fish are introduced into aquaculture containers.
Phage therapy is crucial for reducing mortality due to bacterial infections in aquaculture. Successful application requires careful planning of the timing, dose, and frequency of phage delivery, considering the bacterial pathogen’s virulence characteristics, the nature of the outbreak and the temporal dynamics of the major pathogenic bacteria. In this context, phage therapy is most effective during spring when aquatic pathogenic bacteria exhibit the highest diversity, which correlates with a higher risk of outbreaks. Several factors influence the efficacy of phage therapy in aquaculture, including the fish’s weight, the required phage dose, early disease diagnosis and environmental conditions.
Proteon Pharmaceuticals poised to grow its bacteriophage products for aquaculture
The selection of the phage delivery route depends on the nature of the infection, the size of the farm, the cost of phage preparation, and the fish species. The three primary methods for administering phages in aquaculture are immersion, injection, and oral administration. Immersion is less time-consuming, while injections, which are ideal for systemic infections, are more time-consuming and invasive, potentially causing high mortality. Oral administration is considered the most practical method due to its low cost and minimal stress on the fish.
However, challenges include the loss of phage stability and the acidic, proteolytic conditions in the gut, which may require coating systems or the addition of acid neutralizers to the phage suspension. Bacteriophages should be able to endure dry conditions on feed following coating for oral feed applications. Longer phage survival is critical for reducing feed preparation costs and maximizing commercial benefits.

Phage therapy in fish culture
Aquaculture wastewater and the environment have both been found to contain numerous antibiotic-resistant genes and antibiotic-resistant bacteria. As a result, bacteriophages may be employed as alternatives to antibiotics, helping to reduce antibiotic usage at the source and address concerns related to antibiotics. However, currently, phages are primarily used in research at the bench level or in small-scale trials to prevent and control antibiotic-resistant bacterial infections in aquaculture. Research on the interactions between phages and their aquatic hosts has mainly been conducted in marine environments, with relatively little focus on freshwater ecosystems.
Phage research has focused on some bacterial species belonging to the genera Aeromonas, Vibrio, Edwardsiella, Streptococcus, and Flavovacterium, which are particularly pathogenic for fish, mollusks and crustaceans. Less information is available on other genera, although these pathogens can still cause significant economic damage to the aquaculture industry. Aeromonas spp. is the pathogen for which the highest number of in vivo trials have been performed, followed by Vibrio spp., particularly in larvae models.

Phage therapy in mollusk culture
Bacteriophage therapy may also be effective in treating bacterial infections in mollusk species, with the most targeted bacteria being Vibrio spp., followed by Escherichia coli and Salmonella enterica. The most commonly affected animals are oysters and abalones.
The spread of Vibrio spp. in marine, estuarine, and freshwater environments is responsible for vibriosis, a devastating disease that can cause up to 100 percent mortality in contaminated facilities, not only in fish but also in shellfish. These shellfish can pass the pathogen to humans through the consumption of raw or improperly cooked seafood.
Among the numerous infectious Vibrio species, V. parahaemolyticus is considered one of the primary foodborne pathogens worldwide, while V. coralliilyticus and V. tubiashii are responsible for high mortality rates in oysters, leading to significant economic losses globally. The approach to managing these species varies depending on the goal: for V. parahaemolyticus, research primarily focuses on using phages to purify oysters and ensure their safety for human consumption. In contrast, for V. coralliilyticus and V. tubiashii, phage therapy is aimed at safeguarding the health of oysters and other mollusk species.
Apart from the public health impact, an outbreak of V. parahaemolyticus has significant social repercussions for the entire shellfish-producing region. Additionally, V. parahaemolyticus strains infecting seafood have shown resistance to multiple commercial antibiotics, similar to strains infecting humans, and as a result, the potential of various candidates for bacteriophage therapy has been investigated both in vitro and in vivo.
Phage therapy in crustacean culture

Among bacteria, Vibrio spp. is the leading cause of economic losses in the shrimp industry, followed by Aeromonas spp. All stages of the shrimp life cycle, from eggs to broodstock, can be affected by Vibrio spp. infection, which typically results in 100 percent mortality in contaminated facilities. The genus Vibrio includes a range of pathogenic species that impacts not only the farming of fish and mollusks but also the farming of crustaceans. Therefore, alternatives to disinfectants and the limited number of allowed antibiotics are being explored, with bacteriophage therapy emerging as a promising option. Some phages target multiple Vibrio spp., while others are specific to certain highly pathogenic strains. Among the Vibrio species, the most pathogenic in crustaceans are V. parahaemolyticus, V. harveyi, and V. alginolyticus.
V. parahaemolyticus is regularly monitored in shrimp hatcheries, not only because it is the primary cause of seafood-related bacterial gastroenteritis globally, but also because it causes necrosis, stunted growth, muscle opacity, anorexia, and, ultimately, death in crustaceans. Some strains are responsible for Acute Hepatopancreatic Necrosis Disease, characterized by mortality rates of up to 100 percent in marine shrimp aquaculture.
Since the proportion of a particular microorganism impacts others and the entire microbial community, and given that the gut microbiota of shrimp influences their health, phage therapy may serve as an effective measure to prevent acute hepatopancreatic necrosis disease in the shrimp industry. Furthermore, since acute hepatopancreatic necrosis disease may be caused by various pathogenic strains of V. parahaemolyticus, developing bacteriophage cocktails effective against a broad range of V. parahaemolyticus strains is particularly beneficial.
Several studies have researched the use of varios bacteriophages to manage pathogenic Vibrios (refer to the original publication). These include V. campbellii, is emerging as an opportunistic pathogen associated with luminous vibriosis and potentially linked to acute hepatopancreatic necrosis disease. V. harveyi is a prevalent cause of mortality in hatcheries and culture systems for larval shrimp, in addition to being a pathogen for fish, as mentioned above. V. alginolyticus is another significant pathogenic species that infects a variety of aquatic organisms, including crustaceans, mollusks, fish and humans, where it causes skin and ear infections, as well as acute gastroenteritis.

Perspectives
Phage therapy is increasingly considered the most promising alternative to treat bacterial diseases in cultured aquatic organisms. Aquaculture’s bacteriophage therapy has only gained popularity in recent years, and as a result, the number of studies available is fewer compared to other livestock sectors. The conditions to consider when applying phage therapy in aquaculture are varied and depend on the type of farming. For example, crustaceans have very different requirements compared to fish. Therefore, the stability of phages under certain conditions, such as water salinity, temperature, pH and ultraviolet radiation, is much more stringent.
Most bacteriophages studied have been tested in vivo; however, for those tested only in vitro, it remains impossible to directly translate the results obtained in vitro to an in vivo system. Another issue is that most of the phages used in aquaculture have not been fully characterized in terms of their genomic and proteomic features, so there is limited information on their full potential and possible toxicity to the organism. Indeed, the major limitation to the application of bacteriophage therapy in aquaculture is the lack of comprehensive data, which in turn restricts the genetic engineering of the phages themselves.
Another challenge is the rise of phage-resistant, mutated pathogenic bacterial strains. In this context, cocktails of multiple bacteriophages are the preferred option for combatting multidrug-resistant pathogens. As demonstrated by several comparative studies between single phages and phage cocktails, the latter has proven to be more efficacious in the vast majority of cases. Additionally, the delivery method also impacts on the efficacy of phage therapy.
Administration varies depending on the characteristics of the phage, the nature of the infection, and the animal species. In aquaculture, injection is generally the most effective method for targeting bacteria that cause clinical signs in internal organs, but this method is associated with a high mortality rate. Moreover, when animals are small and numerous, injecting phages can be labor-intensive and time-consuming. Therefore, phage-incorporated diets (phage-coated feeds) have been successfully used to treat large quantities of fish, ensuring continuous delivery of phages at designated times.
Overall, phage therapy as an alternative to antibiotics for treating bacterial diseases in aquaculture is still in the early stages of development. It requires further understanding of the phage susceptibility of virulent bacterial strains, their genetic traits involved in pathogenicity, and the phage–bacterium co-evolution before it can be fully validated.
Now that you've reached the end of the article ...
… please consider supporting GSA’s mission to advance responsible seafood practices through education, advocacy and third-party assurances. The Advocate aims to document the evolution of responsible seafood practices and share the expansive knowledge of our vast network of contributors.
By becoming a Global Seafood Alliance member, you’re ensuring that all of the pre-competitive work we do through member benefits, resources and events can continue. Individual membership costs just $50 a year.
Not a GSA member? Join us.
Authors
-
Deborah Albarella
Department of Veterinary Medicine and Animal Sciences—DIVAS, Università degli Studi di Milano, 26900 Lodi, Italy
-
Paola Dall’Ara
Department of Veterinary Medicine and Animal Sciences—DIVAS, Università degli Studi di Milano, 26900 Lodi, Italy
-
Luciana Rossi
Department of Veterinary Medicine and Animal Sciences—DIVAS, Università degli Studi di Milano, 26900 Lodi, Italy
-
Lauretta Turin
Corresponding author
Department of Veterinary Medicine and Animal Sciences—DIVAS, Università degli Studi di Milano, 26900 Lodi, Italy
Related Posts
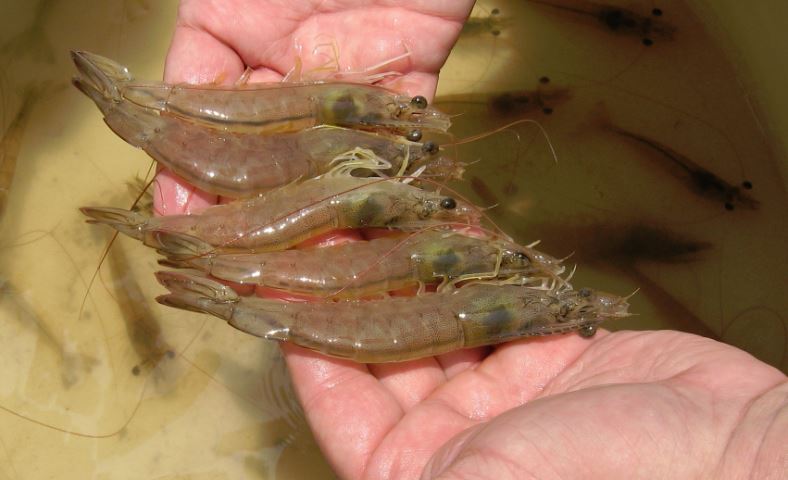
Health & Welfare
Potential applications of bacteriophages for AHPND control
Study demonstrates that isolated phages tested are effective in controlling AHPND infection in farmed penaeid shrimp and inhibiting bacterial growth.
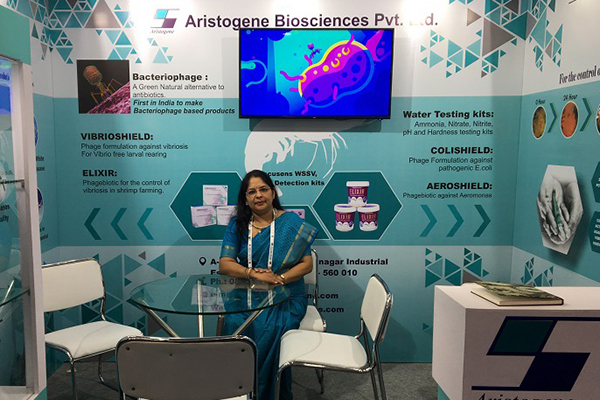
Innovation & Investment
‘Choices are limited when searching for alternatives to antibiotics’: How one veterinarian is employing bacteriophages to fight Vibriosis in shrimp farming
GOAL 2022: Bacteriophages can overcome antibiotics in aquaculture's fight against Vibriosis in shrimp farming, and Dr. C.R. Subhashini is leading that fight.
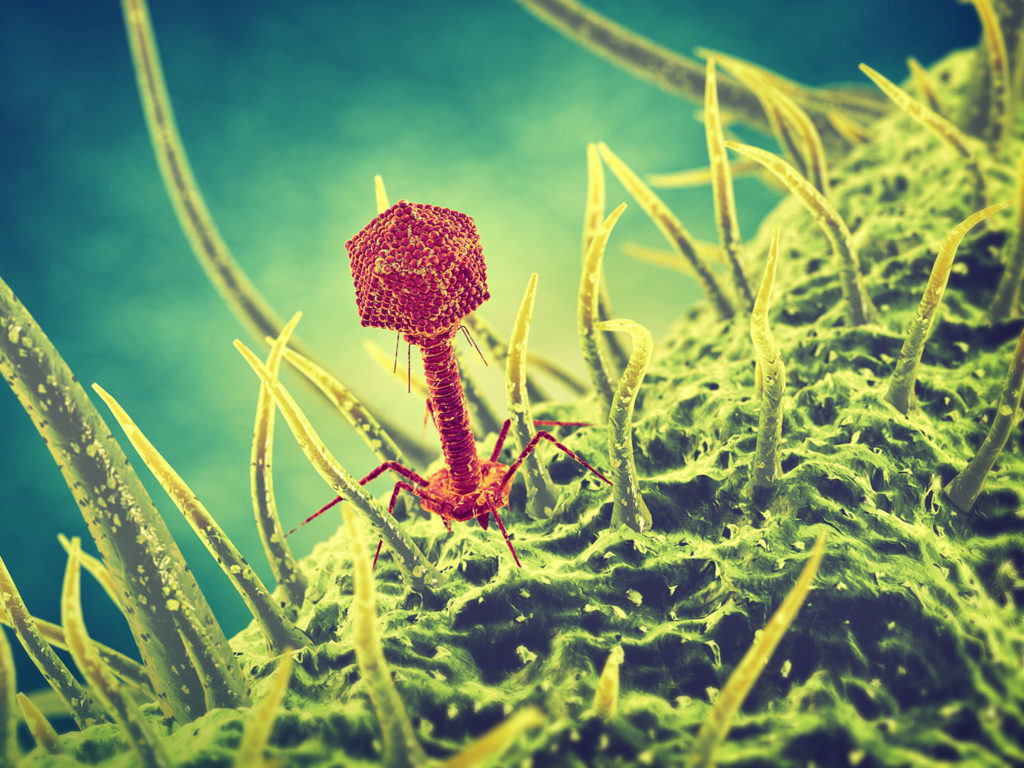
Health & Welfare
Biotech-feed giant partnership to explore bacteriophage potential
Fish health and welfare in aquaculture could soon be assisted by the most abundant organism on the planet, if a new partnership nets its intended result.
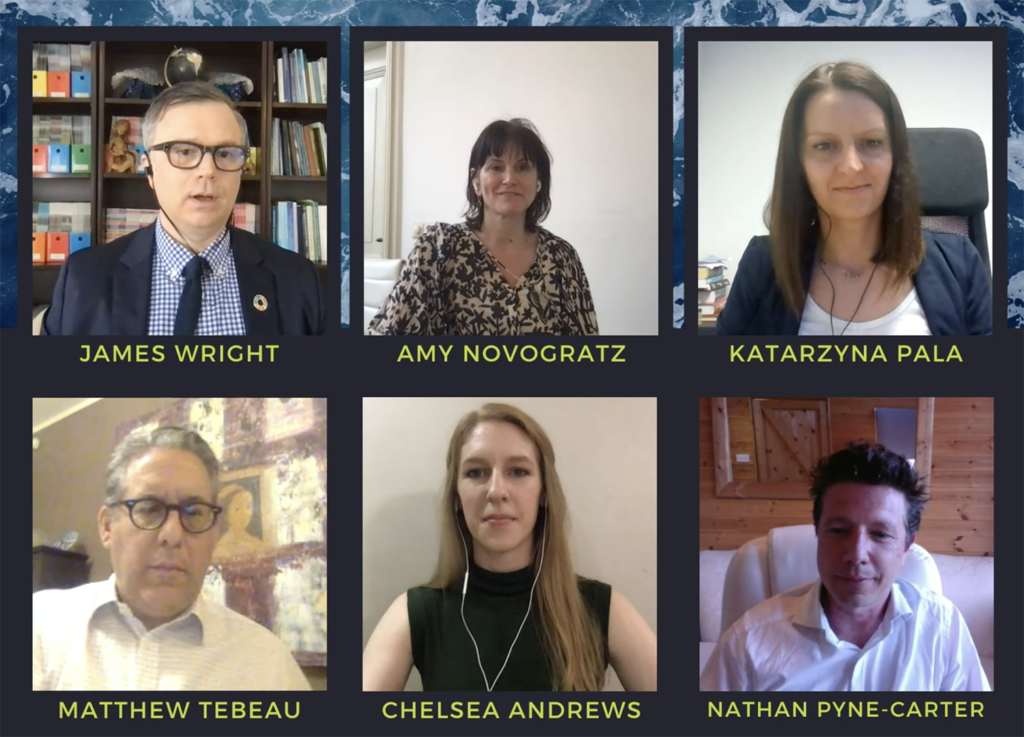
Innovation & Investment
Entrepreneur Tony Fadell joins GOAL 2021 to talk technology and aquaculture
Former Apple and Google developer Tony Fadell told GOAL attendees that new technologies must smooth the pathway to the marketplace.