Project funded by Florida Sea Grant aims to demonstrate the feasibility of a marine aquaponic system
Interest in and development of aquaponic systems has grown rapidly in the last 10 years. Aquaponics offers a unique way to produce fish in a controlled environment while minimizing water use and waste discharge. Aquaponic systems vary in size from small-scale designs appropriate for households to larger commercial operations with yields able to supply farmers markets and high-end restaurants.
Many of the systems in operation are designed to produce freshwater fish and vegetables that grow well hydroponically. While there is great interest in marine aquaponics, development of marine systems has been constrained by limited land-based production of marine fish species and the selection of appropriate edible plant species that grow in saltwater.
Mote Aquaculture Research Park (MAP), the University of South Florida Civil and Environmental Engineering program, and Morningstar Fishermen partnered to design and study a marine aquaponic system. Building on past joint research on an integrated multi-trophic aquaculture system, the goal of the current project funded by Florida Sea Grant is to demonstrate the feasibility of a marine aquaponic system and explore its environmental and economic sustainability.
Species selection
Florida red drum, Sciaenops ocellatus, was selected for the fish species because of its heartiness and ability to tolerate conditions with only 12 to 15 ppt salinity. While harvests of wild-caught red drum have declined in recent years, the species is a popular sport fish, and farm-raised fish can be sold commercially.
Certain species of plants can tolerate low-salinity conditions. However, the use of halophytes, plants that naturally grow in saline conditions, was preferred over forcing freshwater plants to adapt to saline conditions.
Two saltwater vegetable species – sea purslane, Sesuvium portulacastrum; and saltwort, Batis maritima – were selected. Saltwort is a locally grown halophyte similar to the commonly consumed Salicornia species used in European cuisine and as animal fodder. Sea purslane is also native to Florida and has a European counterpart known by similar common names that in fact reflect different species.
Both sea purslane and saltwort are typically harvested from the wild. Research suggests they have numerous health benefits, including high omega-3 fatty acid, vitamin A and vitamin C content. A local nursery that produces both species for coastal restoration projects provided cuttings for the aquaponic system.
System design
Construction began during the summer of 2014, and the system was stocked with red drum fingerlings in September 2014. A schematic of the system is presented in Figure 1. The system consisted of three, 3.3-m3 round fish tanks initially stocked with red drum at a density of 4.23 kg/m3.
Solids were removed first through a swirl separator then through an upflow media filter for fine solids removal. A moving-bed bioreactor was used to ensure enough nitrification capacity and subsequent fish health.
Four plant raceways with dimensions of 12.8 m x 1.2 m were constructed from wood and lined with polyethylene. Cuttings for the two saltwater plant species of 7- to 10-cm length were stocked in the raceways in early October. The saltwater vegetables were hydroponically grown in net pots packed with coconut fiber and supported on polystyrene rafts floating in the raceways. Once water exited the plant raceways, it flowed to a holding tank before being recirculated back to the fish tanks.
Due to the presence of salt in the solids, they could not be discharged to the environment or used as a soil amendment, as commonly practiced in freshwater aquaponics. For this reason, the MAP system was equipped with a sand filter to collect backwash from the radial flow filter and upflow media filter. The solids collected will be used in another project exploring their potential for methane production through anaerobic digestion and as a fertilizer for wetland plants grown for restoration.
Water quality data
During the first three months, water samples were collected weekly from six points throughout the system and analyzed for ammonium-nitrogen and nitrite-nitrogen. Nitrate-nitrogen was analyzed twice weekly. The ammonium-nitrogen concentration fluctuated during the first 50 days, but remained below 1 mg/L (Figure 2). While there was no change in fish feeding behavior, by day 60, the ammonium-nitrogen level rose above 1 mg/L.
Three changes were made to improve ammonia removal: increasing the flow rate, reducing the biomass density and adding alkalinity to improve nitrification. After these changes were made, the ammonium-nitrogen concentration decreased and has not surpassed 0.5 mg/L.
The nitrate-nitrogen concentration increased weekly over the first 90 days of the experiment and did not appear to stabilize. An assessment of the system indicated the sand filter could be modified to improve conditions for denitrification.
Initially, the backwash from the radial flow filter and upflow media filter quickly passed through the sand filter. By partially submerging the sand filter media, anaerobic conditions were created. These conditions, combined with the high nitrate-nitrogen concentration and high levels of organic carbon in the backwash, allowed improved denitrification. Once the changes were made to the sand filter, the nitrate levels decreased.
Plant growth
During the first three months, plant samples were collected twice monthly for later analysis of nitrogen and phosphorus content. The sea purslane grew rapidly and performed well in the aquaponic system (Figure 3). Harvesting of sea purslane began in January. The plants are currently being sold in 0.25-lb (0.11-kg) bunches for U.S. $3.50 at a local famers market and directly to a local restaurant.
The saltwort did not perform as well initially. However, after five months, it appears to be growing better.
Fish growth
The red drum have performed well in the aquaponic system, with a survival rate of 98 percent. The average weight of fingerlings stocked in September 2014 was 46.5 g. On day 100, the average weight was 294.0 g, and the feed-conversion ratio was 1.2. It is anticipated the fish will be ready for harvest late this summer.
Perspectives
According to the Food and Agriculture Organization of the United Nations, the current global food system fails to sufficiently support the nutritional needs of over 870 million people worldwide. Meeting these needs has become increasingly difficult as water scarcity and decreased availability of arable land have begun to constrain agricultural production. Collapsing fish stocks have reduced wild-caught harvests for decades.
Novel methods of food production like marine aquaponics are key to meeting the future nutritional needs of the growing population. This research is just a beginning, and additional work on optimizing marine aquaponic systems and developing markets for the saltwater vegetables will be necessary to ensure the success of this industry.
(Editor’s Note: This article was originally published in the July/August 2015 print edition of the Global Aquaculture Advocate.)
Now that you've reached the end of the article ...
… please consider supporting GSA’s mission to advance responsible seafood practices through education, advocacy and third-party assurances. The Advocate aims to document the evolution of responsible seafood practices and share the expansive knowledge of our vast network of contributors.
By becoming a Global Seafood Alliance member, you’re ensuring that all of the pre-competitive work we do through member benefits, resources and events can continue. Individual membership costs just $50 a year.
Not a GSA member? Join us.
Authors
-
Suzanne Boxman
Doctoral Candidate
Environmental Engineering Program
University of South Florida
4202 East Fowler Avenue, ENB 118
Tampa, Florida 33620 USA[117,100,101,46,102,115,117,46,108,105,97,109,64,115,110,97,109,120,111,98]
-
Kevan Main, Ph.D.
Senior Scientist, Program Manager
Directorate of Fisheries and Aquaculture
Mote Marine Laboratory
Sarasota, Florida, USA -
Michael Nystrom
Senior Biologist
Directorate of Fisheries and Aquaculture
Mote Marine Laboratory
Sarasota, Florida, USA -
Sarina J. Ergas, Ph.D.
Professor
Environmental Engineering Program
University of South Florida -
Maya A. Trotz, Ph.D.
Associate Professor
Environmental Engineering Program
University of South Florida
Related Posts

Intelligence
An engineer’s design for a classroom aquaculture-aquaponics system
An aquaponics teaching system was designed, built and operated by students at the University of Arizona, integrating its operation and management into the educational curriculum. This engineering design will require minimum maintenance and will last years.
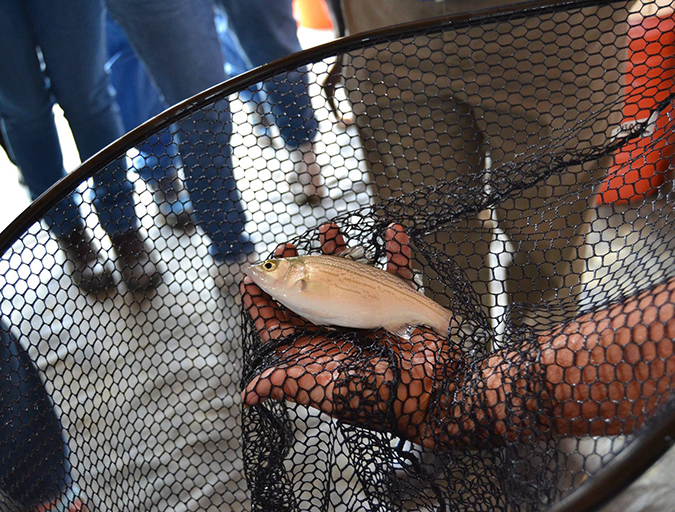
Intelligence
Young aquaponics, aquaculture company gets big boost
Fluid Farms, an aquaponics produce grower in Maine, leans on multi-trophic aquaculture to provide nutrients for its plants. The company is now selling its hybrid striped bass to the local market and, armed with a $50,000 innovation prize that will fund a new heating system, is expanding its horizons.
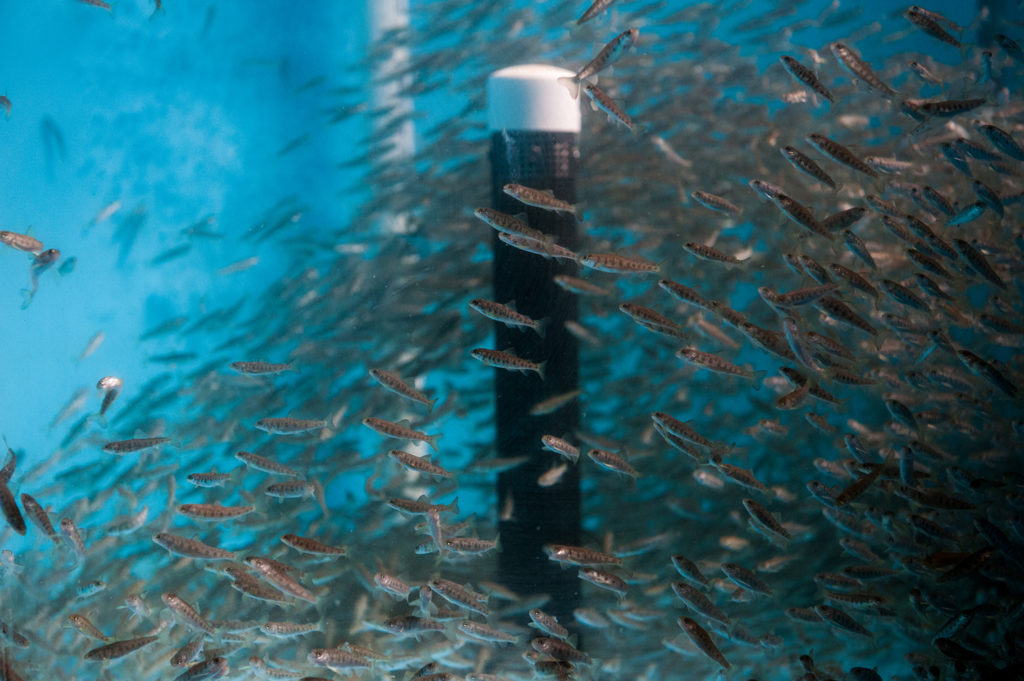
Intelligence
City fish-farm prototype set to prove itself in Minnesota
The Urban Organics model of farming leafy greens in conjunction with fish can work in any city environment, the company says. The first step is destroying East St. Paul’s image as a food desert.
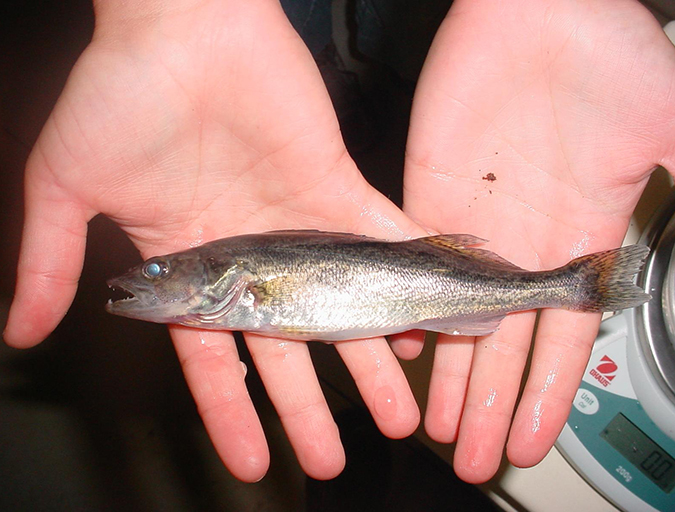
Intelligence
Walleye and saugeye growing successfully indoors
Past and ongoing studies at the UWSP Northern Aquaculture Demonstration Facility (UWSP-NADF) have shown that walleye and hybrid walleye can be successfully raised indoors using early advanced spawning, incubation and rearing techniques. Marketing studies indicate good aquaculture potential and high demand.