Four-year Alabama project improves design, operational efficiency

As world population and seafood demand continue to increase, intensification of aquaculture is unavoidable while fisheries resources, land and freshwater become more limited in many regions. The increase in global trade of aquaculture products also requires more competitive and efficient production approaches by farmers and processors to deliver high quality products to meet global demand.
Freshwater pond aquaculture, in particular, will be further challenged to intensify production, while using less water and zeroing effluents, as government agencies continues to impose more regulation on water uptake and effluent discharge. In addition, consumer awareness on the origin, safety and sustainability of food continues to increase and has driven wholesalers and retailers to impose a cascade of demands for certification of aquaculture products that farmers and processors must meet.
Aquaculture enterprises must move towards more intense and sustainable production strategies, and require more efficient use of water, feed, labor, energy and other resources to maintain high quality products at competitive prices compared to other fish, seafood and animal meats available to consumers.
Aquaculturists are always looking for ways to increase fish yield, as they often associate productivity with increased business competitiveness and profits. However, production in pond aquaculture is limited by the amount of wastes (mainly decaying phytoplankton and metabolic and fecal residues of fish) the biota in a pond can assimilate while maintaining satisfactory water quality for growth and health of fish.
Dissolved oxygen is typically the first limiting factor to fish yield in ponds. Early morning dissolved oxygen in pond water has an inverse relationship to feeding rate, phytoplankton abundance and fish biomass. Pond aeration provides additional oxygen, improves water circulation, reduces water stratification and accelerates the decomposition of wastes. Supplemental aeration, thus, is a worldwide tool farmers use to improve water quality, while increasing feeding rate and fish yield from ponds.
However, in ponds with no water exchange, despite all the aeration that can be supplied, ammonia poisoning is generally considered the second limiting factor of production. Total ammonia concentration increases proportionally to the amount of organic wastes entering the ponds. Thus, feeding rate and decay of dead phytoplankton has a direct relationship to total ammonia levels in pond water.
With more aeration, farmers can stock more fish and add more feed to a pond, targeting higher fish yields. However, this can lead to an excess of organic wastes and nutrients, which favors growth of dense phytoplankton blooms in the ponds. Intense photosynthesis by microalgae can cause pond water pH to reach values above 9.0 at noon and afternoon hours, which increases the risk of unionized ammonia toxicity in heavily fed ponds.
For this reason, in addition to aeration, farmers need to set upper limits to feeding rates, monitor afternoon ammonia and pH levels and apply strategies to prevent excessive phytoplankton. Since water exchange is becoming more restricted in freshwater pond aquaculture, updated pond management and production strategies, in addition to just increasing aeration, are needed to improve water quality to further increase fish yield in heavily fed ponds.
In-Pond Raceway System (IPRS)
The In-Pond Raceway System (IPRS) is a promising strategy to further increase fish yield in static ponds. Instead of growing fish free in the pond, in the IPRS fish are confined at high densities in floating or fixed raceways (Figs. 1 and 2). Water circulation and aeration is continuously provided to each raceway, maintaining adequate and safe oxygen levels in the growing cells, independently of the oxygen status in the pond.
The raceways seldom exceed 3 percent of total pond surface area. The IPRS was first conceived and developed at the School of Fisheries, Aquaculture and Aquatic Science – Auburn University (SFAAS-AU) in the early 1990s. Initially, units were small and constructed with wooden panels. Since then, researchers at SFAAS-AU have conducted several experimental- and commercial-scale evaluations to understanding the potential, advantages and limitations of farming catfish in IPRS compared to conventional or highly aerated catfish ponds. Such evaluations also contributed to improve the design, construction and operation of IPRS, culminating with the semi-commercial size floating raceways made with a metallic frame lined with high density polyethylene (HDPE) and a more efficient airlift device to aerate and circulate the water through the raceways and pond.
Results from the semi-commercial scale evaluation of IPRS at Auburn University
A four-year project is in progress at Auburn University to refine IPRS management protocols, improve design and operational efficiency and assess economic feasibility of semi-commercial scale IPRS to produce catfish. The first year’s goal was to demonstrate that market size catfish (average weight of at least 680 g and minimum weight of 450 g) could be produced in a growing period of 8 to 10 months, at a yield twice the average yield of 7,800 kg/ha attained in conventional catfish ponds in Alabama.
Four 0.4-ha earthen ponds were each equipped with an IPRS (Figure 2). The IPRS units in B1 and B2 were 63.6 m3 in volume (4.9 m wide, 10.7 m long and 1.2 m water depth), while in B3 and B4 smaller units of 45.3 m3 were used (3.1 m wide; 12.2 m long and 1.2 m water depth). Each IPRS pond was supplied with 2.5 HP of aeration and water circulation effected by two regenerative air blowers.
One 1.5-HP blower propelled the air lift apparatus at the entrance of the IPRS raceway, while another 1.0-HP blower propelled the air lift apparatus of the water moving unit installed at a pond corner diagonally opposite from the IPRS unit. A 55-m long and 1.5 m high baffle curtain, made of woven plastic fiber, was installed diagonally inside each pond to direct the water circulation around the entire pond.

The raceways were stocked with 41-g hybrid catfish fingerlings (female channel catfish Ictalurus punctatus x male blue catfish I. furcatus) on March 22, 2016. Fish were fed 32 percent crude protein commercial floating catfish pellets (4 to 6 mm) once or twice a day, depending upon water temperature. Each feeding event lasted for 3 to 5 minutes, until near momentary satiation of fish.
Dissolved oxygen, temperature and other pond water parameters were regularly monitored. Catfish reached market size in early December 2016. Raceways were harvested after nearly 270 days of culture (Figure 3), and fish were sold to an Alabama catfish processor. A summary of the production results is presented in Table 1.

Kubitza, Raceway, Table 1
Pond B1 | Pond B2 | Pond B3 | Pond B4 | |
---|---|---|---|---|
Raceway volume (m3) | 63.6 | 63.6 | 45.4 | 45.4 |
No. of fish stocked | 11,030 | 11,086 | 8,083 | 7,821 |
Feed used (kg) | 9,699 | 9,817 | 8,200 | 7,733 |
Harvested biomass (kg/raceway) | 6,388 | 6,601 | 5,510 | 5,467 |
Final mean weight (g) | 617 | 794 | 712 | 817 |
Pond gross yield (kg/ha) | 15,971 | 16,502 | 13,774 | 13,666 |
FCR | 1.64 | 1.60 | 1.59 | 1.50 |
Standing crop in raceways (kg/m3) | 100.5 | 103.9 | 121.4 | 120.4 |
Survival (%) | 86.4 | 75.0 | 95.7 | 85.6 |
Water quality variable: | Pond B1 | Pond B2 | Pond B3 | Pond B4 |
Dissolved oxygen inside raceway (range in ppm) | 2.2 – 9.2 | 2.4 – 9.8 | 1.9 – 9.2 | 1.8 – 9.9 |
Afternoon water p (ranTotal alkalinity (range as ppm CaCO3) | 100 to 117 | 68 to 78 | 52 to 72 | 80 to 84 |
Afternoon water pH (range) | 7.0 - 9.5 | 7.0 - 8.0 | 7.0 - 9.0 | 7.0 - 9.5 |
Maximum afternoon TAN (mg/L) | 4.80 | 8.00 | 4.80 | 1.80 |
Maximum afternoon NH3 (mg/L) | 1.66 | 0.50 | 0.34 | 0.01 |
Maximum NO2- (mg/L) | 1.50 | 1.50 | 1.60 | 0.80 |
Most predominant water color | Green | Light brown | Green | Green |
Most common algae bloom | SBG (1) | No blooms | SBG (1) | SBG (1) |
Secchi disk at summer and early fall (m) | 0.12 – 0.28 | 0.35 – 0.66 | 0.16 – 0.32 | 0.15 – 0.38 |
(1) SBG – surface blue green (cyanobacteria) blooms
Production, feeding rate and water quality
Catfish yield ranged from 13,660 to 16,500 kg/ha and exceeded the target value of 15,600 kg/ha in ponds B1 and B2. Average feeding rates ranged from 70 to 90 kg/ha/day. Maximum feeding rates of 300 to 350 kg/ha/day was reached in all the ponds early fall (late September, in USA), when fish already weighed more than 550 g. Dissolved oxygen concentrations were often low at early morning hours during summer months in all the ponds.
However, inside the raceways oxygen was seldom below 3 mg/L. Dissolved oxygen close to 2 mg/L inside the raceways were registered a few days, when dissolved oxygen in the open pond water declined to values around 1 mg/Liter, as can be seen in Figure 4 for pond B3, the pond that had the lowest oxygen levels.
Other parameters of water quality are summarized in Table 1. Maximum levels of total ammonia nitrogen (TAN) were 1.8 mg/L in pond B4 and as high as 8.0 mg/L in Pond B2. Fish were exposed to the highest concentration of unionized ammonia (N-NH3 = 1.66 mg/L) in pond B1, since the afternoon water pH in that pond often reached values around 9.0 and 9.5 due to the presence of dense phytoplankton blooms.
In pond B2, despite the high total ammonia levels, toxic ammonia levels were not a concern at all, since phytoplankton blooms did not become established in that pond to cause pH to increase (afternoon pH ranged from 7.0 to 8.0 in pond B1). Nitrite concentration in all ponds remained well below the 7 mg/L LC50-96h determined for channel catfish.
Nonetheless, pond preparation protocol included the application of salt (NaCl) to prevent nitrite toxicity of fish. Chloride levels in pond water ranged from 100 to 140 ppm for all ponds, except for water in B1, which had 300 ppm of chloride.
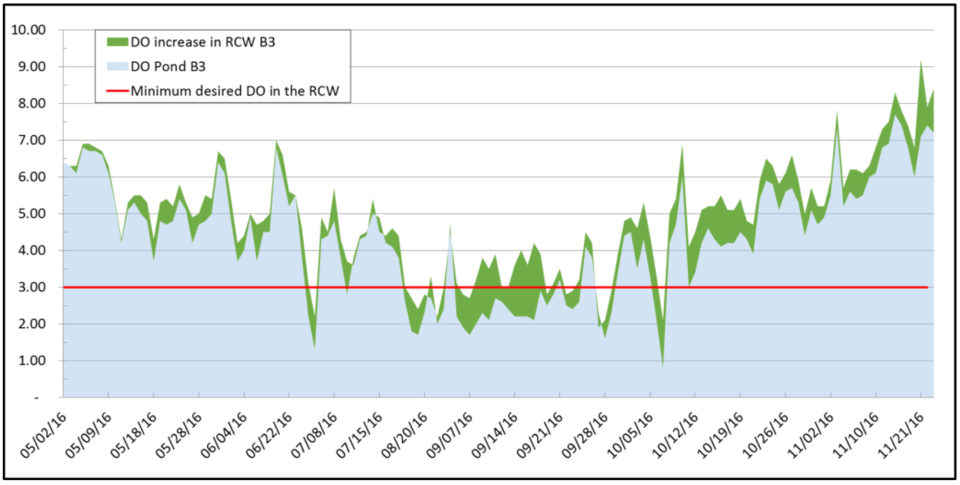
Authors
-
Fernando Kubitza, Ph.D.
Invited Researcher (corresponding author)
Auburn University
School of Fisheries, Aquaculture and Aquatic Sciences
Auburn AL 36849-5419 USA[117,100,101,46,110,114,117,98,117,97,64,54,48,48,48,107,122,102]
-
Jesse A. Chappell, Ph.D.
Associate Professor / Extension Specialist
Auburn University
School of Fisheries, Aquaculture and Aquatic Sciences
Auburn AL 36849-5419 USA[117,100,101,46,110,114,117,98,117,97,64,49,106,112,112,97,104,99]
-
Terrill R. Hanson, Ph.D.
Professor / Extension Specialist
Auburn University
School of Fisheries, Aquaculture and Aquatic Sciences
Auburn AL 36849-5419 USA[117,100,101,46,110,114,117,98,117,97,64,114,116,110,111,115,110,97,104]
-
Esau Arana
Research Associate IV
Auburn University
School of Fisheries, Aquaculture and Aquatic Sciences
Auburn AL 36849-5419 USA[117,100,101,46,110,114,117,98,117,97,64,115,101,97,110,97,114,97]
Tagged With
Related Posts
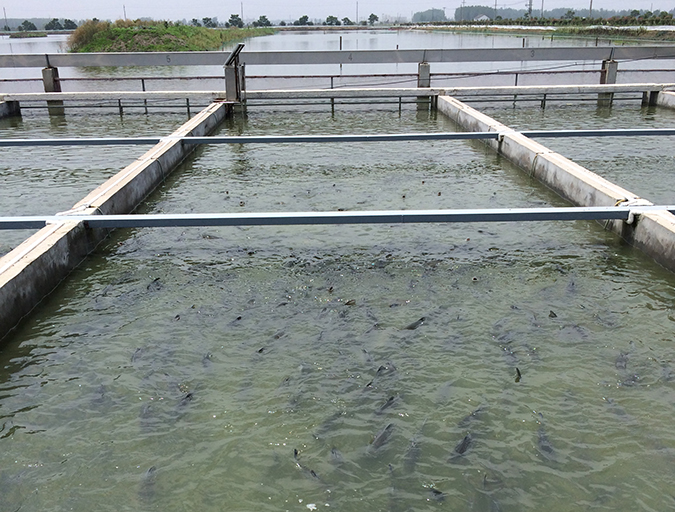
Innovation & Investment
GOAL 2016 preview: IPA technology catching on in China
Intensive pond aquaculture (IPA) technology, a floating, in-pond raceway system developed in the United States, is being adopted fast in China, just three years after its introduction, says Jim Zhang, aquaculture program manager for USSEC-China.
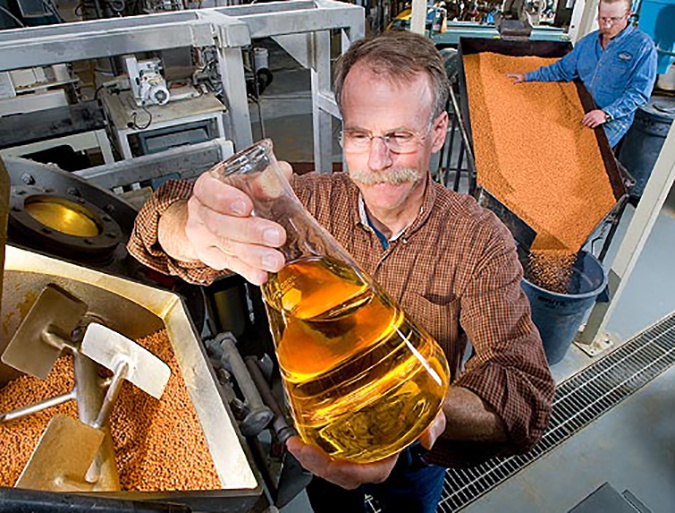
Aquafeeds
Aquaculture Exchange: Rick Barrows
After 14 years with the USDA’s Agricultural Research Service, Rick Barrows talks about the importance of finding ‘complete’ and commercially viable alternative sources of omega-3 fatty acids and continuing innovation in the aquafeed sector.
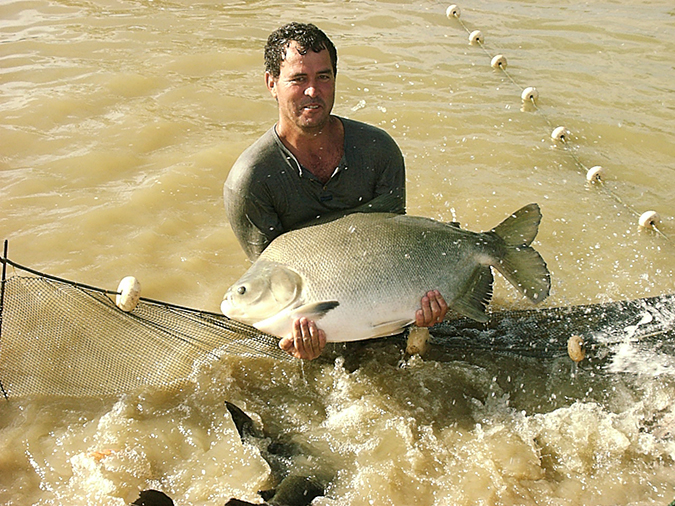
Intelligence
Brazilian aquaculture: Constraints and challenges (Part 1)
The Brazilian aquaculture industry has been growing steadily during the last two decades. Despite facing a number of challenges it is looking at continued growth and a larger role in the export markets.
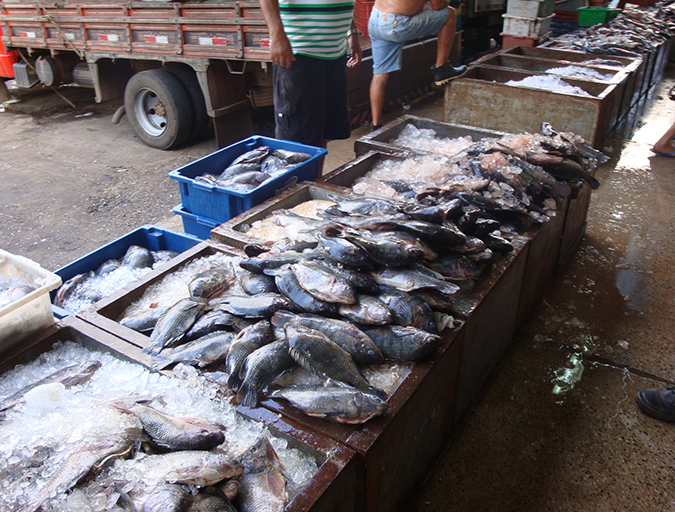
Intelligence
Brazilian aquaculture: Constraints and challenges (Part 2)
Brazil will have to deal with an adverse economic and political environment in the next few years. One should expect high value fish products like shrimp, tilapia, Chilean salmon and cod being replaced by more affordable seafood and alternative meats, as consumers keep losing purchasing power due to inflation, unemployment and monetary devaluation.